Charging Ahead: The Future of Batteries
Battery research is at a tipping point, and it’s never been more important
/https://tf-cmsv2-smithsonianmag-media.s3.amazonaws.com/filer/a5/65/a565aadd-0dc3-4584-8a03-9a6038646edf/future-of-batteries.jpg)
The world is waiting on a battery breakthrough. Nearly every sector of the electronics industry, everything that runs on a battery, is limited by the power output and energy life of the batteries that run it.
“The progress or the advancement of batteries is much slower than in other fields, and this is an intrinsic limitation of batteries,” says Stefano Passerini, editor-in-chief of the Journal of Power Sources. “You cannot expect a battery that can supply energy to a cell phone for a week or a month. At the very end, the maximum amount of energy you can store in a battery is fixed by the available elements.”
But there is progress. Researchers are working on improving the energy density (juice per weight and volume), the price, the safety, environmental impact, and even the lifetime of the most popular class, lithium-ion batteries, as well as designing whole new types.
Most batteries can be found in three main industries: consumer electronics, automotive, and grid storage.
“I would call them the three big buckets of where people intersect with batteries,” says Venkat Srinivasan, deputy director of research and development at the Department of Energy’s Joint Center for Energy Storage Research. Each bucket has different requirements, and thus the batteries used can (sometimes) be very different from each other. That phone in your pocket needs a battery that is compact and safe, but the weight and cost are less important. Scale up to automotive batteries, and with so many batteries, cost and weight become important, as well as cycle life (you’d be very mad if that new Tesla required new batteries every couple of years). Scale up even further, and the batteries that are beginning to be used to store power for houses and the grid have very little weight or size requirements.
For decades, consumer electronics—your phone, computer, camera, tablet, drones, even your watch—have run on lithium-ion batteries, thanks to their easy rechargeability and high energy density. In these batteries, a lattice of graphite, stuffed with lithium ions, forms the anode. An oxide forms the cathode, connected to the opposite terminal, and the two are separated by a liquid electrolyte that allows ions to pass through it. When the external terminals are connected, the lithium oxidizes and the ions flow to the cathode. Charging is just the reverse. The more lithium ions that can be transferred this way, the more power the battery can hold. We’ve come to appreciate the compact size and ease of use, if not the battery life and safety. But there may not be a lot of room for further improvement, says Passernini.
“Now lithium-ion batteries are kind of close to the limit,” he says. “Although we were already saying this about 10 years ago, and the improvements in the last 10 years have been quite substantial.”
In the case of cars, batteries are ultimately responsible for the lifetime of the car and for the dreaded range anxiety when it comes to electric cars. To tackle this problem, engineers and scientists are trying to cram more voltage capacity into batteries. But that’s often associated with faulty chemical reactions, which diminish the capacity over time. A great deal of research is devoted to finding new materials and chemicals to assist or replace the lithium-ion lattice, or other parts of the battery.
Srinivasan points out a couple potential innovations, and these are not just for cars only: The traditional graphite anode lattice could be replaced with silicon, which holds 10 times as many lithium ions. But silicon tends to expand as it absorbs lithium, so batteries will have to account for that. Or: Instead of the lattice, lithium metal could act as the anode—provided we can figure out how to keep it from catastrophically shorting out when it is recharged. It’s an issue that battery manufacturers have been trying to solve since the lithium-ion battery was invented decades ago. “We’ve gotten very hopeful that we’re at a time when maybe this 30-year-old problem can be addressed again,” says Srinivasan.
Perhaps lithium could be replaced entirely. Researchers are looking at ways to use sodium or magnesium instead, and the Joint Center for Energy Storage Research is using computer modeling to investigate custom-designed, oxide-based materials that could work as the cathode to a magnesium anode. Magnesium is especially attractive because its structure allows it to accept two electrons per atom, doubling the charge it can hold.
Prashant Jain and his collaborators at the University of Illinois are working on a different facet of lithium batteries: the electrolyte. The electrolyte is the fluid that fills the space between the cation (positively charged ion) and anion (negatively charged ion), allowing charged particles to flow through. It’s long been known that certain solid materials, like copper selenide, will also allow ions to flow, but not quickly enough to run high-powered devices. Jain, assistant professor of chemistry, and his students, have developed a superionic solid, made from nanoparticles of copper selenide, that has different properties. It allows charged particles to flow at a rate comparable to a liquid electrolyte.
The potential benefits of this technology are twofold: safety and life cycle. If a current lithium-ion battery gets damaged, the battery shorts and heats up. The liquid vaporizes, and nothing is there to prevent a rapid discharge of energy—boom. A solid will prevent that short and allow a full-metal anode, which offers a greater energy capacity. Additionally, over repeated cycles, liquid electrolytes begin to dissolve the cathode and anode, and this is a primary reason that the batteries eventually fail to charge.
“There have been all these incremental improvements that have actually made some advances. But there’s never been a big dramatic breakthrough, disruptive technology where one can say now, the solid electrolyte really matches up to the potential in terms of transporting ions that liquid electrolytes [can],” says Jain. “Now that the safety issues are coming to the fore, with liquid electrolytes, researchers have been like, maybe we need to think of something dramatic with solid electrolytes and, once and for all, make one that can replace a liquid electrolyte.”
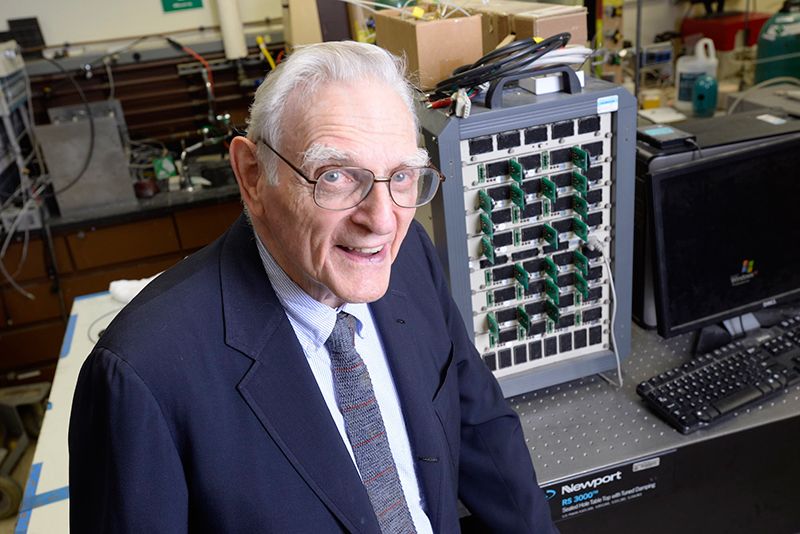
One of the co-inventors of the original lithium-ion battery himself is taking another tack toward solid-state electrolytes: John Goodenough, emeritus professor of engineering at the University of Texas, has published and filed a patent application for a battery with a glass-based electrolyte. By impregnating the glass with lithium or sodium, Goodenough has been able to allow the current to flow even faster while preventing shorts and increasing energy capacity with a solid anode.
All this research is going to be influential to the batteries in our pockets and automobiles. But there’s a third category, where the impacts are global.
Melanie Sanford is using modeling tools on a different type of battery—huge, redox flow batteries that will store power from renewable power plants and release it when the wind and sun aren’t available. Evening out the peaks and valleys of energy production and consumption will help renewables scale up to provide more than just supplementary power.
Southern California Edison is already experimenting with battery banks, using Tesla car batteries, but because the batteries are traditional lithium ion-based, they’re too expensive to use on a scale that will allow global renewable power. Besides, the constraints for a grid battery are much different than a car. Weight and size are not an issue, but price and lifetime are.
In a redox flow battery, energy storage material is held in liquid form in large tanks, then pumped to a smaller cell where it reacts with a similar apparatus that has the opposite charge. The computer modeling has allowed Sanford’s lab to custom design organic molecules, leading to a thousand-fold increase, from less than a day to months, in the amount of time these molecules remain stable.
“For the grid-scale source, the kind of thing you need is materials that are super cheap, because we are talking about huge batteries,” says Sanford. “We are talking about a wind farm, and then a comparable area of warehouses holding these batteries.”
According to Sanford, innovations will come both from materials science—developing new materials to put in our batteries—and from engineers who will make the systems built around those materials more efficient. Both will be needed, but the pipeline from research to production will necessarily be another bottleneck.
“Everybody should be aware that there is not one battery that can fit all applications,” says Passerini. “It’s clear that even to gain a little bit—10 percent, 20 percent performance—it’s a big issue. We need to do research in the field. The scientists need to be supported.”