Ancient Spacedust Reveals Surprising Twist in Evolution of Earth’s Early Atmosphere
Mini fossil meteorites are providing new insights about how our ancient atmosphere evolved into its current state
/https://tf-cmsv2-smithsonianmag-media.s3.amazonaws.com/filer/b4/69/b469fdaa-cecc-4f57-becc-5c2c23002093/for-media-12.jpg)
Specks of ancient spacedust that drifted to Earth 2.7 billion years ago are giving scientists their first glimpse into the chemical makeup of our young planet’s upper atmosphere.
The research suggests Earth’s ancient upper atmosphere contained about the same amount of oxygen as it does today, about 20 percent. That flies in the face of what scientists had assumed: Since the lower atmosphere of the early Earth was low in oxygen, researchers thought the upper atmosphere was similarly devoid of the gas.
Scientists say the findings, detailed in this week’s issue of the journal Nature, opens up a new avenue for investigating atmospheric evolution in deep time and provides fresh insight into how Earth’s atmosphere evolved into its current state.
“The evolving atmosphere changed the chemistry of a large range of geological processes, some of which are responsible for forming gigantic mineral resources," says lead study author Andrew Tomkins of Monash University in Melbourne, Australia. So this research "helps us think about biosphere-hydrosphere-geosphere interactions and how they’ve changed over time,” he explains.
The spacedust, or “micrometeorites,” used for the study were recovered from ancient limestone samples from the Pilbara region in Western Australia. The cosmic spherules melted after entering the Earth’s atmosphere at altitudes of about 50 to 60 miles.
“People have found micrometeorites in rocks before, but nobody had thought to use them to investigate atmospheric chemistry,” Tomkins says.
As the tiny objects melted and reformed high up in the ancient atmosphere, they reacted with the oxygen in their surroundings and were transformed. The researchers were able to peer into these ancient micrometeorites to see what chemical changes they had undergone during their trip through the atmosphere.
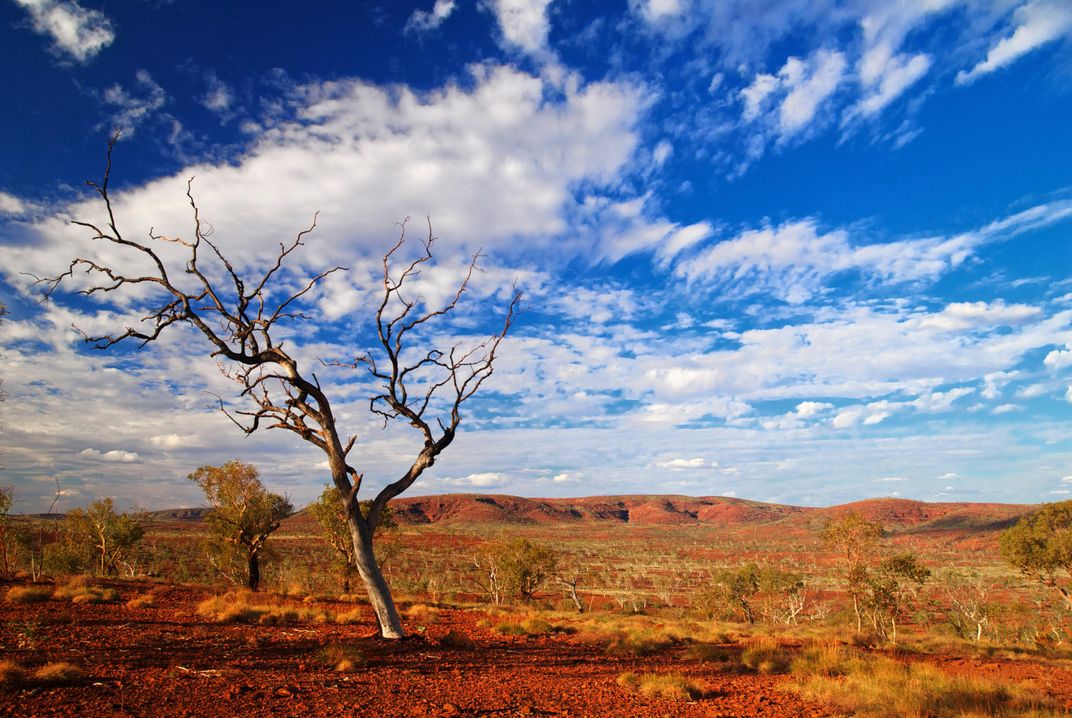
With the aid of a microscope, Tomkins and his colleagues found that the micrometeorites had once been particles of metallic iron that had turned into iron oxide minerals after being exposed to oxygen.
The scientists argue that in order for such a chemical transformation to occur, oxygen levels in the Earth’s upper atmosphere during the Archean Eon (3.9 to 2.5 billion years ago) must have been much higher than previously thought.
Calculations performed by study coauthor Matthew Genge, a cosmic dust expert at Imperial College London, suggest that the oxygen concentration in the upper atmosphere would need to be approximately 20 percent — or close to modern day levels — to explain the observations.
“I think it’s really exciting that they possibly have a way of testing [upper] atmospheric composition through these micrometeorites,” says Jim Kasting, a geoscientist at Pennsylvania State University who was not involved in the study.
Tomkins and his team think their new results could support an idea proposed by Kasting and others that Earth’s atmosphere during the Archean was stacked, with the lower and upper atmospheres separated by a hazy middle layer. That layer would have been composed of the greenhouse gas methane — produced in large quantities by early methane-producing organisms, called “methanogens."
The methane would have absorbed ultraviolet light and released heat to create a warm zone that blocked the vertical mixing of different atmospheric layers.
According to this scenario, the haze layer would have inhibited vertical mixing until the “great oxidation event” 2.4 billion years ago, when photosynthesizing cyanobacteria produced oxygen in large enough quantities that it could dispel the methane.
“Oxygen and methane don't go well together, so this rise in oxygen would have eventually reacted the methane out of the system,” Tomkins says. “Removal of methane would allow more effective mixing of the upper and lower atmospheres.”
Tomkins stressed, however, that this hypothesis still needs to be tested, and he has plans to team up with Kasting to develop computer models to simulate vertical mixing in atmospheres with different compositions.
“We have taken a sample of the upper atmosphere at only a single point in time,” Tomkins says. “The next step is to extract micrometeorites from rocks covering a broad range of geological time, and to look at broad changes in the chemistry of the upper atmosphere.”
Learn more about this research and more at the Deep Carbon Observatory.