Can Evolution Make the Next Generation of Computer Chips?
In a recent experiment, genetic mutation and artificial selection were harnessed to make semiconductors
/https://tf-cmsv2-smithsonianmag-media.s3.amazonaws.com/filer/20120628023050Intel_Pentium_Processor_backside_with_heat_sink-small.jpg)
In 1965, Intel co-founder Gordon Moore made a prediction about computing that has held true to this day. Moore’s law, as it came to be known, forecasted that the number of transistors we’d be able to cram onto a circuit—and thereby, the effective processing speed of our computers—would double roughly every two years. Remarkably enough, this rule has been accurate for nearly 50 years, but most experts now predict that this growth will slow by the end of the decade.
Someday, though, a radical new approach to creating silicon semiconductors might enable this rate to continue—and could even accelerate it. As detailed in a study published in this month’s Proceedings of the National Academy of Sciences, a team of researchers from the University of California at Santa Barbara and elsewhere have harnessed the process of evolution to produce enzymes that create novel semiconductor structures.
“It’s like natural selection, but here, it’s artificial selection,” Daniel Morse, professor emeritus at UCSB and a co-author of the study, said in an interview. After taking an enzyme found in marine sponges and mutating it into many various forms, “we’ve selected the one in a million mutant DNAs capable of making a semiconductor.”
In an earlier study, Morse and other members of the research team had discovered silicatein—a natural enzyme used used by marine sponges to construct their silica skeletons. The mineral, as it happens, also serves as the building block of semiconductor computer chips. “We then asked the question—could we genetically engineer the structure of the enzyme to make it possible to produce other minerals and semiconductors not normally produced by living organisms?” Morse said.
To make this possible, the researchers isolated and made many copies of the part of the sponge’s DNA that codes for silicatein, then intentionally introduced millions of different mutations in the DNA. By chance, some of these would likely lead to mutant forms of silicatein that would produce different semiconductors, rather than silica—a process that mirrors natural selection, albeit on a much shorter time scale, and directed by human choice rather than survival of the fittest.
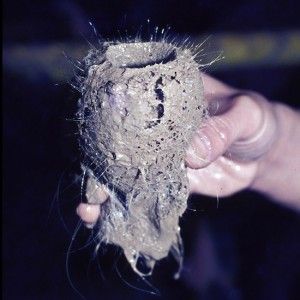
To figure out which mutated forms of the silicatein DNA would lead to the desired semiconductors, the DNA needed to be expressed through a cell’s molecular machinery. “The problem was that, although silica is relatively harmless to living cells, some of the semiconductors that we want to produce would be toxic,” Morse said. “So we couldn’t use living cells—we had to use a synthetic surrogate for cells.” As an artificial replacement for cells, the team used tiny bubbles of water formed around plastic beads. A different form of the marine sponge DNA was attached to each of the millions of beads, and the chemicals necessary for the DNA to be expressed as an enzyme were included in the water.
Next, the plastic bead “cells” were encased in oil, which acted as an artificial cell membrane. The beads were then put in a solution that included the chemicals (silicon and titanium) needed for the mutant enzymes to start building semiconductor minerals on the outside of the beads.
After allowing some time for the enzymes to do the work of making minerals, the beads were passed through a laser beam, next to a sensor that automatically detected when either of the desired semiconductors (silicon dioxide or titanium dioxide) passed through. Afterward, the successful beads—those that had these semiconductors accumulated on their outsides—were broken open so the mutant DNA could be isolated and its effect could be confirmed.
Various forms of silicon dioxide are currently used in the production of computer chips, while titanium dioxide is used in manufacturing solar cells. The production of substances like these using biological enzymes and directed evolution is a first.
While this certainly doesn’t mean that the researchers had cells pumping out computer chips, it does point to a new method of creating semiconductors. The semiconductors made by the mutant enzymes in the experiment, Morse said, “have never before been produced in nature, and have never before been produced by an enzyme, but they’re presently used in industry for all kinds of communications and information processing.” A few years down the road, new and specialized forms of semiconductors produced using this method could even play a role in ensuring Gordon Moore’s prediction stays true.