Seven Simple Ways We Know Einstein Was Right (For Now)
For the past 100 years, these experiments have offered continued evidence that general relativity is our best description of gravity
/https://tf-cmsv2-smithsonianmag-media.s3.amazonaws.com/filer/8b/99/8b99c1a1-054d-4845-ab4f-50f89d7e9e20/15861603283_3579db3fc6_o.jpg)
For 100 years, Albert Einstein's general theory of relativity has survived just about every test that physicists have thrown at it. Announced in November 1915, the famous scientist's field equations expanded on Isaac Newton's long-standing laws by re-imagining gravity as a warping in the fabric of space and time, rather than a simple force between objects.
The results of using general relativity equations actually look similar to what you get using Newton's math, as long as the masses involved aren't too large and the velocities are relatively small compared to the speed of light. But the concept was a revolution for physics.
Warped space-time means that light itself is affected by gravity much more strongly than Newton predicted. It also means that planets move around their orbits in a slightly altered but very significant way, and it predicts the existence of exotic objects such as monster black holes and wormholes.
General relativity isn't perfect—the rules of Einstein's gravity seem to break down when you apply them to the rules of quantum mechanics, which reign at subatomic scales. That leaves plenty of tantalizing gaps in our understanding of the universe. Even today, scientists are pushing the limits to see how far relativity can take us. In the meantime, here are a few of the ways we consistently see relativity in action:
Mercury's Orbit
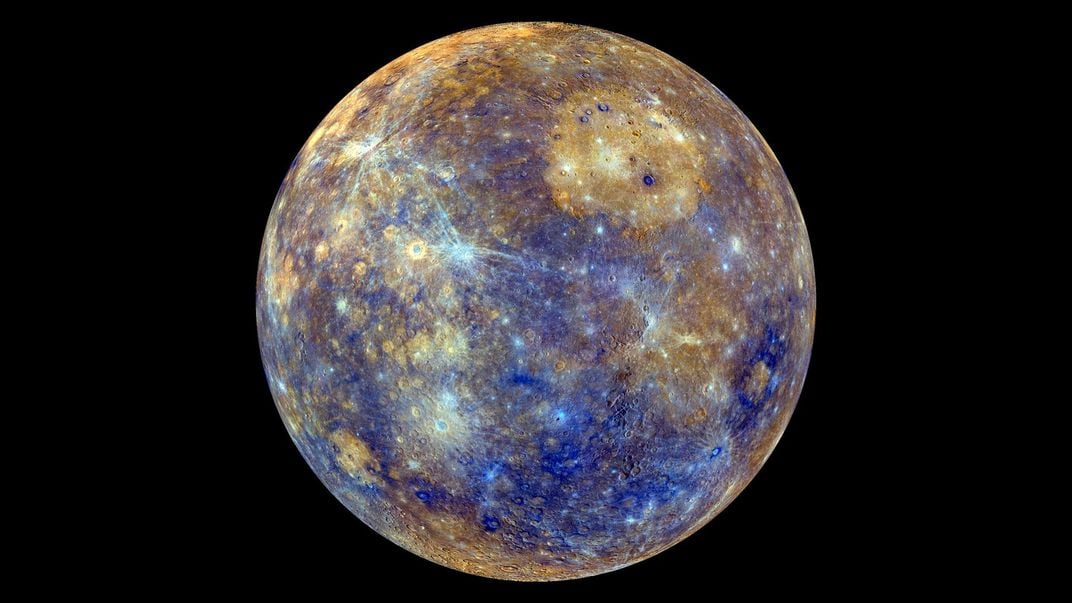
Back in the 19th century, astronomer Urbain LeVerrier noticed a problem with Mercury's orbit. Planetary orbits are not circular, they are ellipses, which means that planets can be closer or farther from the sun and from each other as they move through the solar system. As planets tug on each other, their points of closest approach move in a predictable fashion, a process called precession.
But even after accounting for the effects of all the other planets, Mercury seemed to be precessing a teeny bit further than it should each century. At first astronomers thought that another, unseen planet dubbed Vulcan must be inside Mercury's orbit, adding its gravitational pull to the mix.
But Einstein used the equations of general relativity to show that no mystery planet was needed. Mercury, being closest to the sun, is simply more affected by the way our massive star curves the fabric of space-time, something Newtonian physics did not account for.
Bending Light
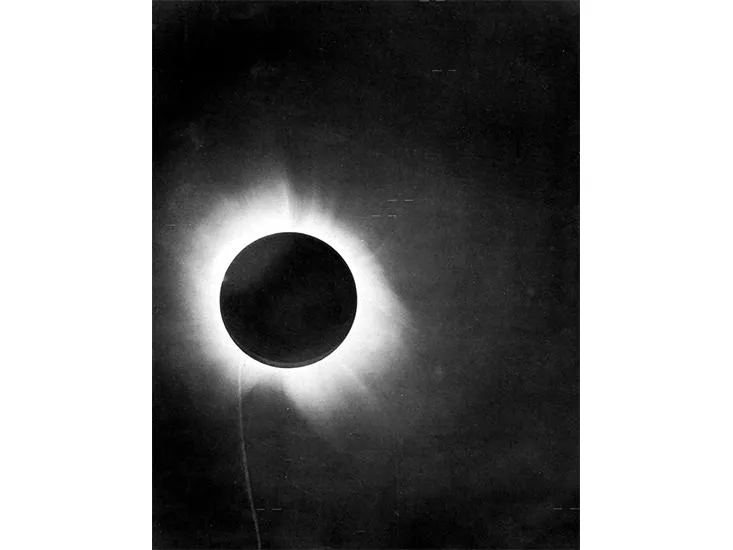
According to general relativity, light moving through the fabric space-time should follow the curves of that fabric. That means light moving around massive objects should bend around them. When Einstein published his general relativity papers, it wasn't clear how to observe this distortion, since the predicted effect is small.
British astronomer Arthur Eddington hit on an idea: look at the stars near the edge of the sun during a solar eclipse. With the glare of the sun blocked by the moon, astronomers could see if the apparent position of a star was changed as the massive sun's gravity bent its light. The scientists made observations from two locations: one in eastern Brazil and one Africa.
Sure enough, Eddington's team saw the displacement during a 1919 eclipse, and newspaper headlines trumpeted to the world that Einstein was right. In recent years, new examinations of the data have shown that by modern standards the experiment was flawed—there were problems with the photographic plates, and the precision available in 1919 wasn't actually good enough to show the right amount of deflection in the measurements from Brazil. But subsequent experiments have shown the effect is there, and given the absence of modern equipment, the work was solid enough.
Today astronomers using powerful telescopes can see the light from distant galaxies being bent and magnified by other galaxies, an effect now called gravitational lensing. This same tool is currently used to estimate the masses of galaxies, to look for dark matter and even to seek out planets orbiting other stars.
Black Holes
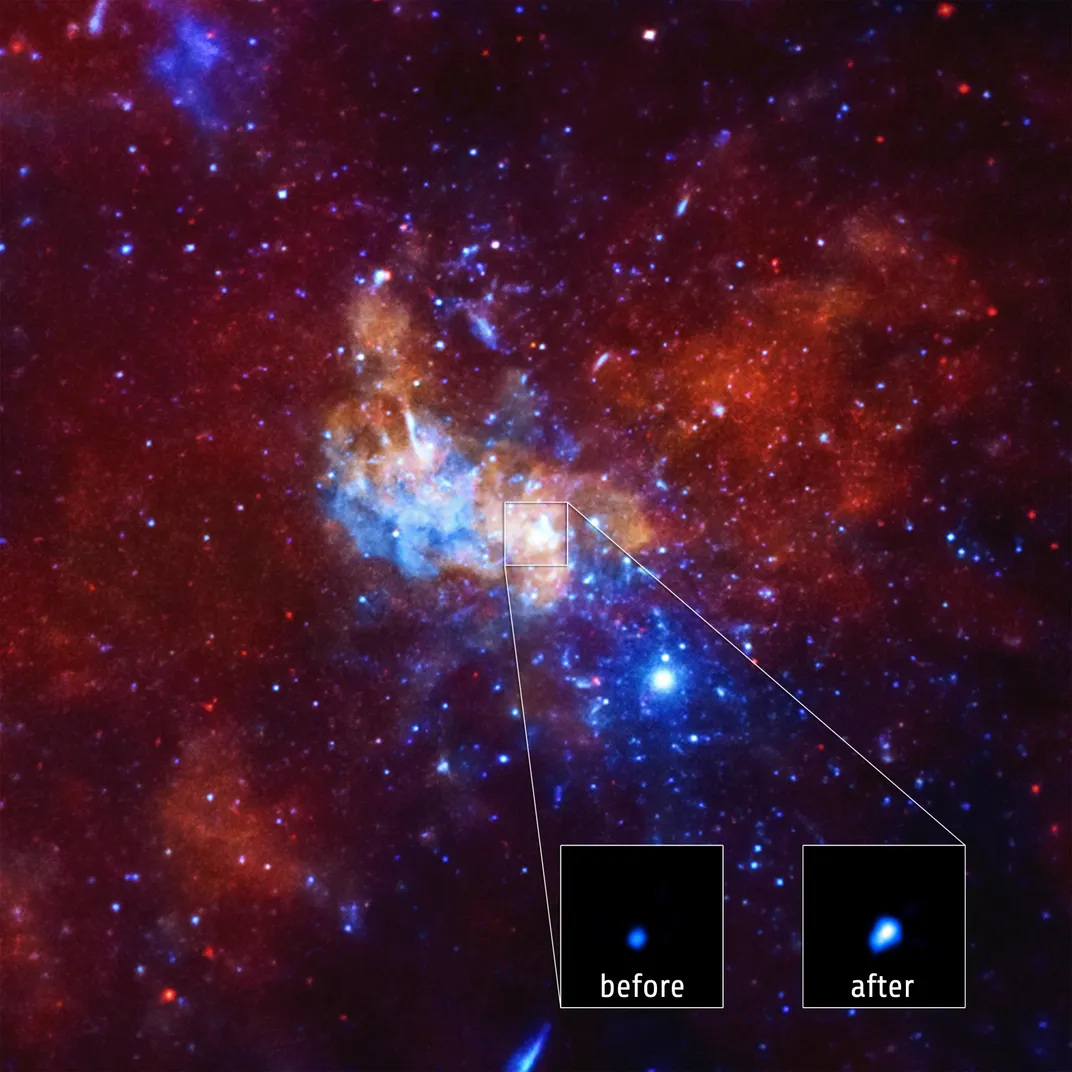
Perhaps the most spectacular prediction of general relativity is the existence of black holes, objects so massive that not even light could escape their gravitational pull. The idea, however, was not new. In 1784, an English scientist named John Mitchell presented it at the Royal Society meetings, and in 1799 Pierre-Simon LaPlace, a French mathematician, arrived at the same concept and wrote a more rigorous mathematical proof. Even so, nobody had observed anything like a black hole. In addition, experiments in 1799 and afterwards seemed to show light must be a wave rather than a particle, so it wouldn't be affected by gravity the same way, if at all.
Enter Einstein. If gravity is actually due to a curvature of space-time, then it could affect light. In 1916 Karl Schwarzschild used Einstein's equations to show that not only could black holes exist, but that the resulting object was almost the same as LaPlace's. Schwarzschild also introduced the concept of an event horizon, a surface from which no material object could escape.
Though Schwarzschild's mathematics were sound, it took decades for astronomers to observe any candidates—Cygnus X-1, a strong source of X-rays, became the first object widely accepted as a black hole in the 1970s. Now astronomers think every galaxy has a black hole at its core—even our own. Astronomers carefully traced the orbits of stars around another bright X-ray source in the center of the Milky Way, Sagittarius A*, and found that the system behaves like an extremely massive black hole.
"For systems like Cygnus X-1 or Sagittarius A*, we can measure the mass and the radius of the compact object, and we simply can't figure out any other astrophysical object that would have the same observational properties," says Paul M. Sutter, an astrophysicist and a visiting scholar at Ohio State University.
Shooting the Moon
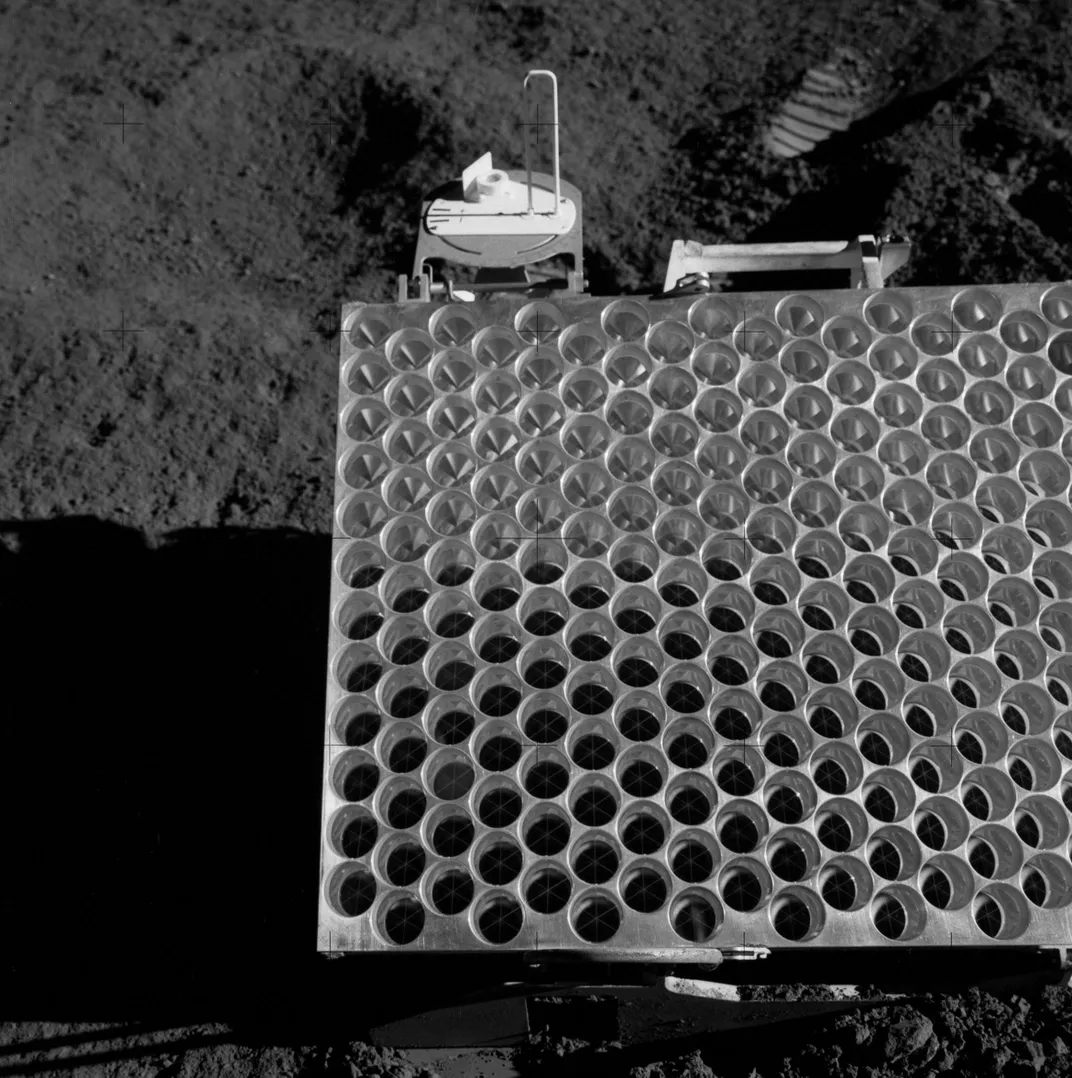
In crafting his general theory of relativity, Einstein realized that the effects of gravity and the effects of acceleration are both caused by the curvature of space-time, and that the gravitational force experienced by someone standing on a massive object would be akin to the effect experienced by someone accelerating away, say, by riding on a rocket.
That means the laws of physics as measured in a laboratory will always look the same no matter how fast the lab is moving or where it is in space-time. Also, if you put an object in a gravitational field, its motion will depend only on its initial position and its velocity. That second statement is important, because it implies that the tug of the sun's gravity on Earth and the moon should be very stable—otherwise, who knows what trouble might ensue if our planet and the moon "fall" toward the sun at different rates.
In the 1960s, the Apollo missions and Soviet lunar probes set up reflectors on the moon, and scientists on Earth have been firing laser beams at them to run a host of scientific experiments, including measuring the distance between Earth and the moon and their relative motions around the sun. One of the lessons from this lunar range-finding was that Earth and the moon are indeed falling toward the sun at the same rate, just as general relativity predicts.
Dragging Space
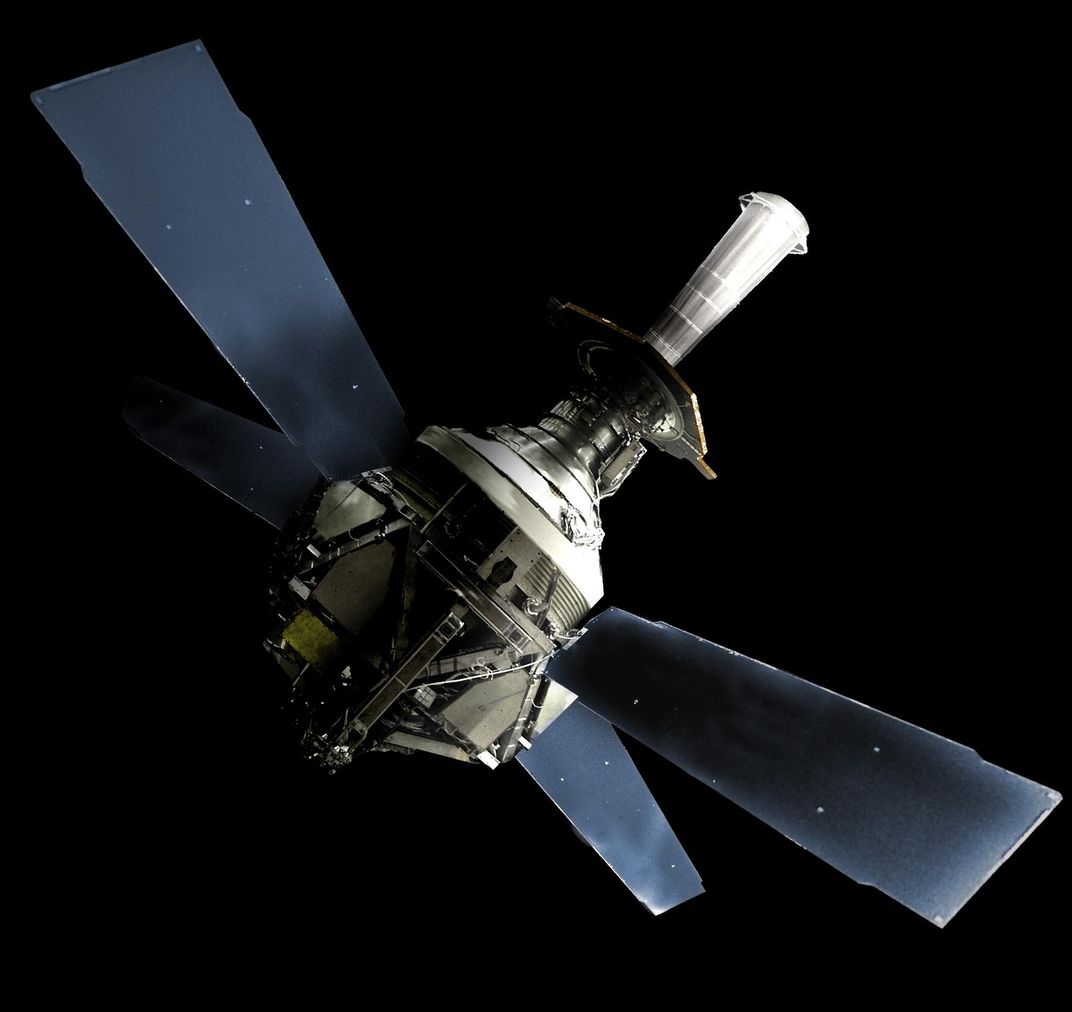
In most descriptions of general relativity, people imagine Earth as a bowling ball suspended on a piece of fabric, aka space-time. The ball causes the fabric to distort into a depression. But since Earth rotates, general relativity says that depression should twist and distort as the ball spins.
A spacecraft called Gravity Probe B, launched in 2004, spent a year measuring the curvature of space-time around the Earth. It found some evidence for frame-dragging, or the Earth dragging the cosmic fabric with it as it rotates, helping to validate Einstein's picture of gravity.
Space-Time Ripples
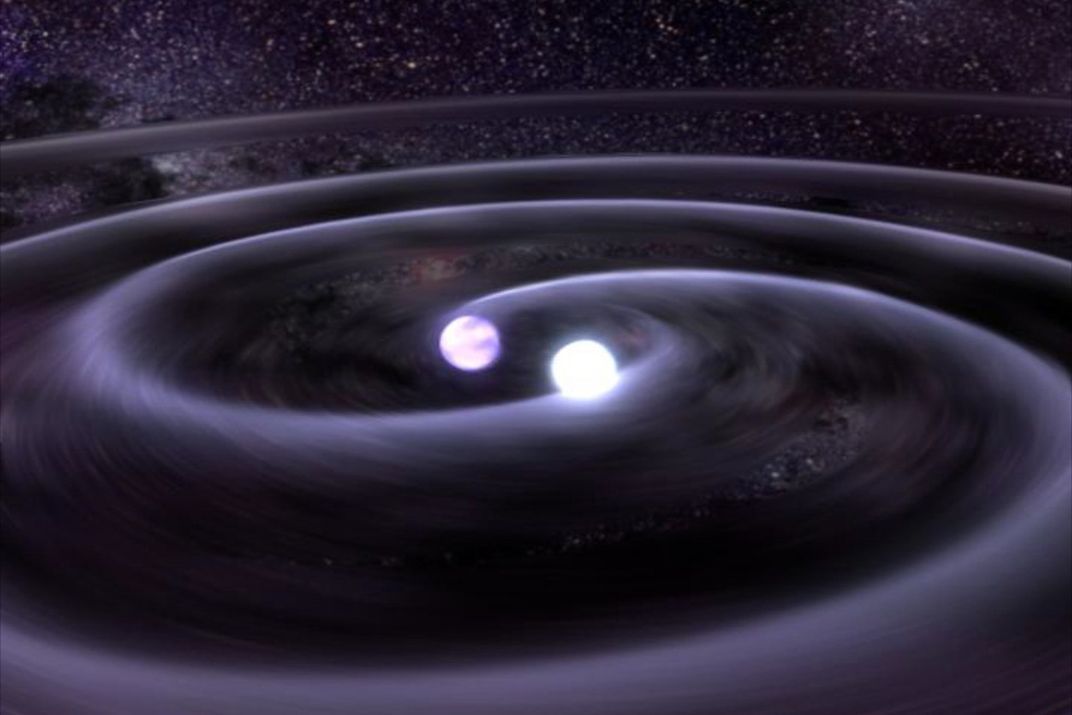
Another consequence of objects moving through space-time is that sometimes they will create ripples and waves in the fabric, akin to the wake of a ship. These gravitational waves would stretch space-time in ways that theoretically are observable. For example, some experiments shine a laser beam between two sets of mirrors and time how long it takes the beam to bounce between them. If a space-time ripple passes through Earth, such detectors should see a tiny lengthening and contraction of the beam, which would show up as an interference pattern.
So far, gravitational waves are one of the last major predictions of general relativity that have yet to be seen, though there are rumors of a detection at a facility in the U.S. But there is some indirect evidence. Pulsars are dead stars that pack many times the mass of the sun into a space the size of Manhattan. Observations of two pulsars orbiting each other provide some hints that gravitational waves are real.
"The orbital period of the first binary pulsar has been observed to decay over time by about 0.0001 seconds per year," says physicist Alan Kostelecky of Indiana University. "The rate of decay matches the energy loss due to gravitational radiation that is predicted by general relativity."
GPS
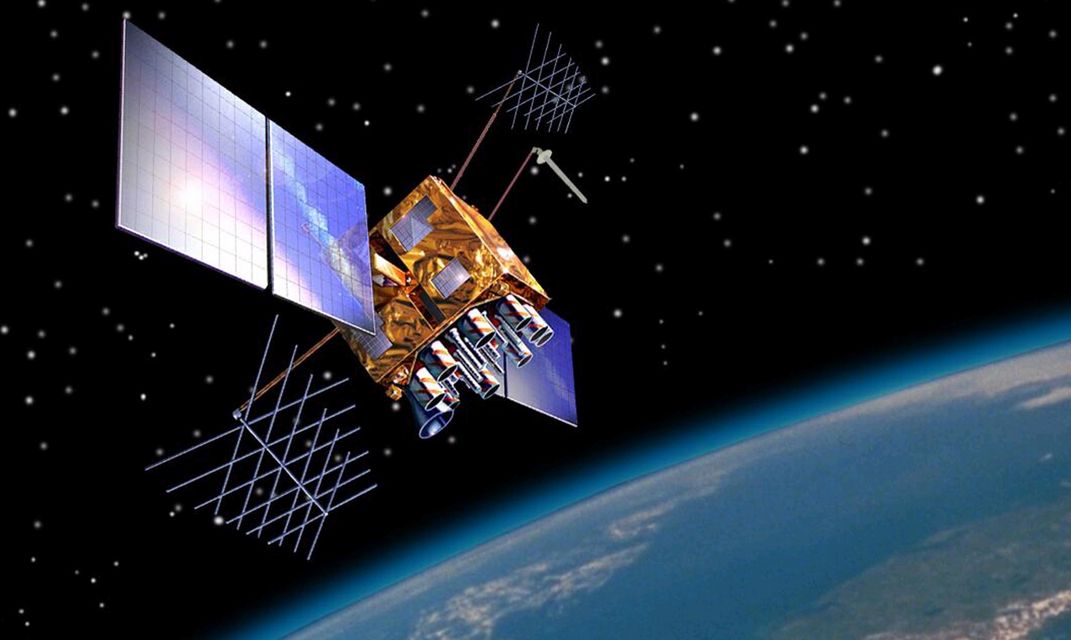
Global Positioning Systems are not exactly a test of relativity, but they absolutely rely on it. GPS uses a network of orbiting satellites that ping signals to phones and rented cars all over the planet. To get a position, those satellites have to know where and when they are, so they keep time measurements to an accuracy of billionths of a second.
But the satellites are circling 12,550 miles above our heads, where they feel less of the planet's gravitational pull than people on the ground. Based on Einstein's theory of special relativity, which says that time passes differently for observers moving at different speeds, the satellite clocks tick a bit slower than the watch on an earthbound traveler.
However, general relativity helps to cancel out this effect, because gravity close to Earth's surface slows down the ticks of a clock as compared to the satellite speeding overhead. Absent this relativistic combo, GPS clocks would be off by about 38 microseconds per day. That may sound like a small error, but GPS requires such high accuracy that the discrepancy would make your mapped location noticeably wrong in a matter of hours.