For the World’s Wetlands, It May Be Sink or Swim. Here’s Why It Matters
One of the world’s most long-studied marshes has revealed a wealth of information, but it continues to perplex and intrigue the scientists who analyze it
/https://tf-cmsv2-smithsonianmag-media.s3.amazonaws.com/filer/88/7d/887da6c9-d9b0-4d3b-b2ce-c9265d95943d/gcrewatsunrise2tommozdzerweb.jpg)
For 30 years, scientists have been watching a salt marsh in central Maryland breathe. That is, they’ve been studying how one Chesapeake Bay ecosystem draws carbon dioxide from the atmosphere, stores some of the carbon underground and releases some of it back into the air in the form of methane gas.
Along the way they’ve manipulated the environment to mimic a future world with more atmospheric carbon dioxide (CO2), the greenhouse gas most responsible for global warming, higher sea levels and more nutrients in the water from polluted runoff. When the growing season begins this spring, they’ll be exploring yet another piece of the puzzle in hopes of gaining a clearer picture of what the future holds. They want to know what happens to the marsh when temperature rises.
“We’ve been raising CO2 in this marsh for 30 years, but [elevated] CO2 comes with warming,” says Pat Megonigal, lead researcher of the new study in the Global Change Research Wetland at Smithsonian Environmental Research Center (SERC). “Warming air translates over time into the soil. We’re just getting around to attacking that part of it.”
As the deputy director at the Environmental Research Center, Megonigal oversees this field site where dozens of scientists conduct experiments. Here the marsh is littered with test plots that look like clear plastic rooms built over patches of reeds and grasses. The plastic contraptions dot a landscape crisscrossed by boardwalks, cables and hoses. Here and there, the boardwalks are punctuated by wooden boxes that house the various control stations.
Researchers like Megonigal have been studying climate change in this 125-acre marsh in an undeveloped patch of the Rhode River for more than three decades. What they’ve learned has important implications, not only for the future of wetlands, but also for impending climate change, because losing wetlands such as marshes and bogs could release millions of tons of carbon dioxide into the atmosphere.
Despite taking up just four to six percent of the Earth’s land area, wetlands such as marshes, bogs and mangrove forests hold a quarter of all the carbon stored in the Earth’s soil.
All plants take up carbon dioxide from the atmosphere and turn the carbon into leaves, stems and roots. But the carbon is released back into the atmosphere when bacteria in the soil decompose fallen leaves and other dead plant material.
In a wetland, however, frequent inundation with water deprives the oxygen-loving bacteria of oxygen and slows them down. Dead plant material doesn’t decay as quickly as it would in a drier environment, so it accumulates, compacting and turning into carbon-rich peat. Storing carbon in this way buffers the atmosphere from rising carbon dioxide.
But there’s a darker side to the story. The soggy wet conditions are primed for fermentation, which produces methane, another carbon-based greenhouse gas that is 25 to 45 times more potent than carbon dioxide. In fact, wetlands constitute the largest single source of methane, producing an estimated 22 percent of all global methane emissions.
In December 2015, leaders from 195 countries hashed out an agreement in Paris limiting global warming to no more than 2 degrees Celsius (3.6 degrees Fahrenheit) above pre-industrial levels. In addition, they committed to pursuing methods that would reduce that number to 2.7 degrees Fahrenheit above pre-industrial levels.
Averaged over the entire globe, temperatures have already risen 1.4 degrees F in the past 120 years, so achieving such ambitious goals will require a rapid reduction in global greenhouse gas emissions, something that can’t be monitored without a reasonably accurate accounting of the balance between carbon emissions and carbon storage throughout the world. For that, global leaders have to understand what’s going on in the wetlands.
“Nothing can be taken off the table,” says Virginia Burkett, chief scientist for climate and land use change at the U.S. Geological Survey. “All systems will have to be assessed in terms of their ability to store carbon, not just emissions alone. The sequestration of carbon, and how humans can enhance the ability of systems like wetlands to store carbon is also essential to understand, in order to make these tremendous reductions that are projected, and expected, and committed to by the international community.”
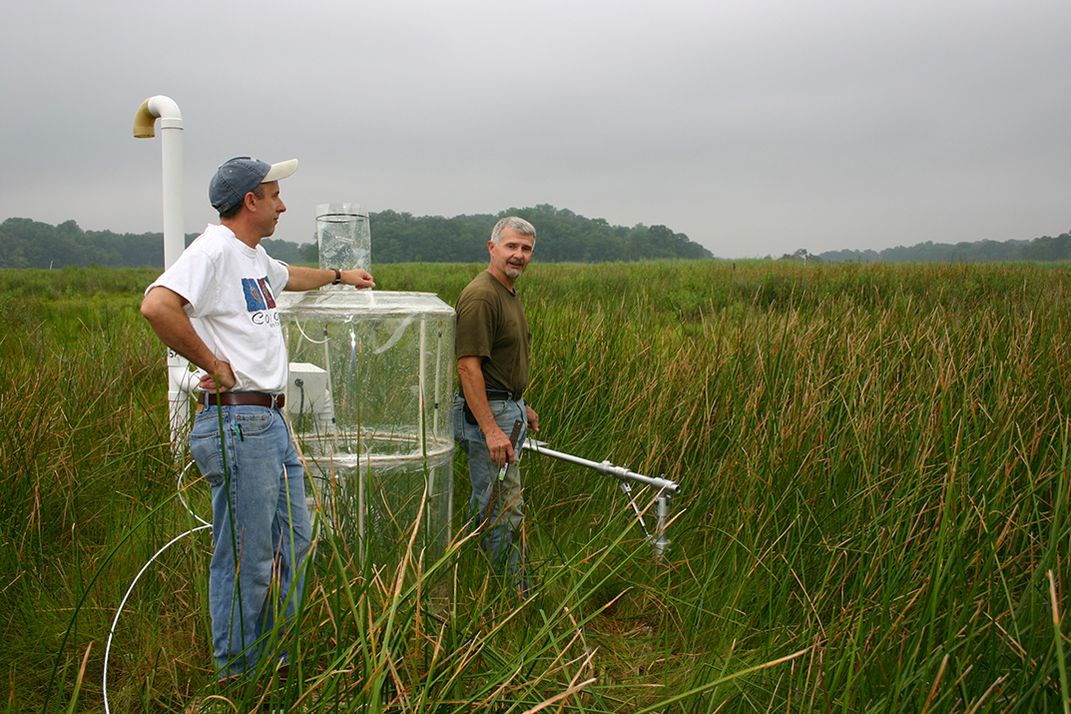
Factoring natural ecosystems into the equation, however, isn’t going to be easy.
How much carbon wetlands take up, how much they release, how quickly soil accumulates and whether tidal wetlands will keep pace with or be swallowed by rising seas are all factors that are intertwined with one another and dependent upon a variety of influences.
Like the tugging of one line in a tangled web of ropes, as one loop loosens, another tightens, changing the shape of the whole bundle. In a marsh, temperature, salinity, carbon dioxide and pollution running off the land are all changing at once. Over the years, scientists have been picking at the knot, unraveling the complexities, but there is far more to understand.
As Megonigal’s soil warming experiment gets going this spring, he’ll be cranking up the heat from the top of the plants all the way down to the bottom of the root zone, four and a half feet below the surface.
By spring, his team will have added 30 new test plots to their corner of the marsh. Using a bank of infrared heat lamps and a grid of electric cables sunken into the soil, Megonigal will raise the temperature in his plots in steady increments. The increase will range from 0 degrees to up to 7.2 degrees Fahrenheit above the surrounding environment, approximating the warmest conditions predicted for the year 2100 if nothing were done to curb climate change.
His primary goal is to understand the factors influencing decay and accumulation of dead plant matter in the salt marsh. If the peaty soil builds quickly enough, it may be able to keep pace with sea level rise. If not, the marsh may simply drown.
The question is a nail-biter for communities that depend on marshes, which provide nursery grounds for important commercial fish, and buffer low-lying land from storm surge and battering waves.
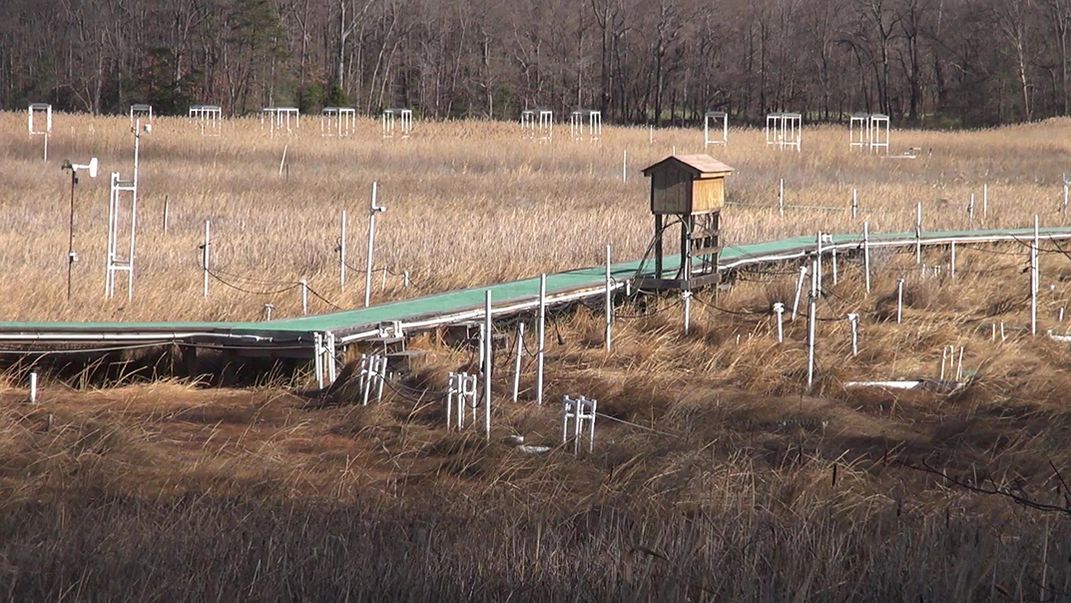
According to soil cores, the salt marsh at Environmental Research Center has survived for 4,000 years. Over that time, the Chesapeake Bay has risen 15 feet, and the marsh has built itself up steadily to keep pace.
Many wetlands around the world have done the same. But the climate is changing and sea level is rising faster than ever. In addition, pollution has changed the chemistry of the water and newly introduced species of plants and animals may be altering important aspects of the way the ecosystem functions. Even the amount of sediment washing into wetlands has changed rapidly with human development on the land.
Megonigal anticipates the added heat will rev up the microbes underground, increasing the rate at which the roots and other organic matter decompose. If so, it could presage the slow sinking of the marsh and the release of more methane into the atmosphere. Then again, maybe not.
Perhaps slower microbes will begin to dominate,” says Stephen Long, professor of crop science and plant biology at the University of Illinois and chief editor of the journal Global Change. Or the combination of warming and added carbon dioxide will cause plants to grow faster than they can decay, both of which might raise the level of the marsh. “It becomes very difficult to predict with any certainty what’s going to happen, which is why an experiment like this is so important,” he says.
Long is among the many researchers who have conducted experiments at the Smithsonian marsh site. He says the very thought of doing this type of work in the natural environment was revolutionary when the first experiment was established 30 years ago. There are so many factors that have to be controlled or accounted for in nature that many in the scientific community thought it couldn’t be done.
Bert Drake, a plant ecologist and senior scientist emeritus at Environmental Research Center, is the man who proved them wrong back in 1985.
A plant’s growth correlates to the amount of carbon it takes in, and Drake initially devised an elegant experiment to monitor growth in the marsh. “I said well, instead of going out there and measuring all the plants, we’ll just measure the CO2 flux,” he says. “People who reviewed our proposal thought we were extending ourselves well beyond what they believed was workable in the laboratory into the field.”
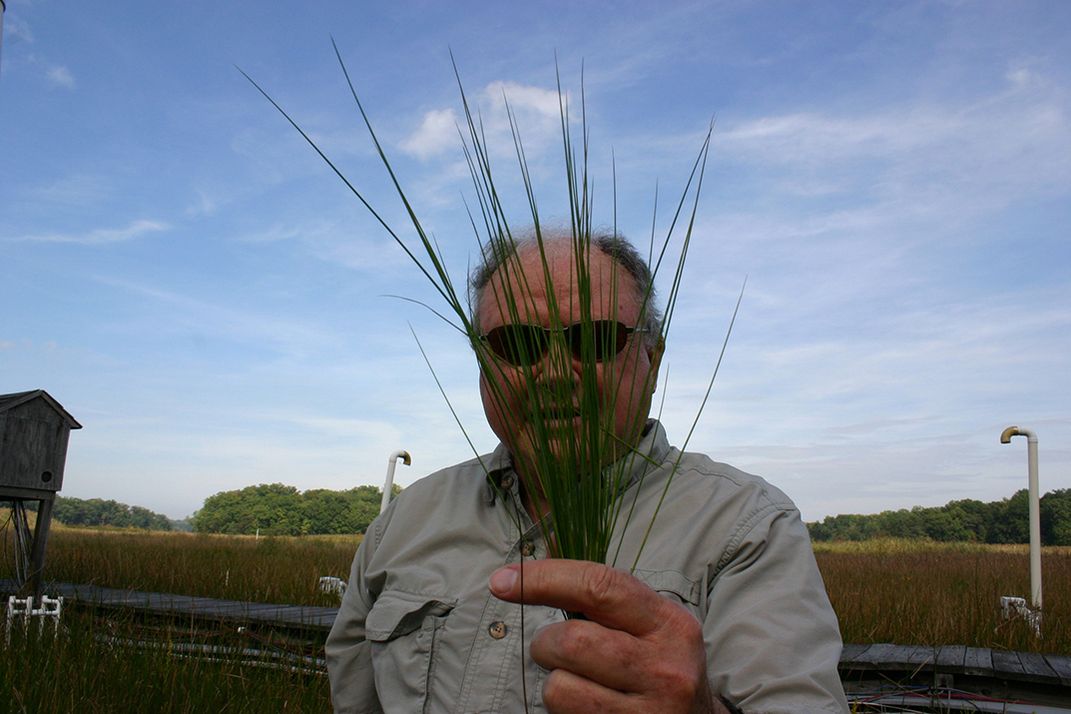
Drake designed a series of open-bottom, cylindrical chambers to place over patches of marsh. About three feet in diameter, they had an octagonal aluminum pipe frame with clear plastic walls and an open top so they wouldn’t trap heat like a greenhouse. He then piped carbon dioxide into the chambers, raising the level to what was expected 100 years in the future.
“We could monitor the concentration of the CO2 going into the chambers, and the CO2 inside, and the CO2 going out,” he says. Immediate results showed that the sedges in Drake’s chambers grew with added vigor, readily soaking up the additional carbon dioxide, while the grasses didn’t change. The pattern matched what scientists had seen in the laboratory and proved his method worked. He had successfully managed a controlled study in an otherwise uncontrollable environment. Drake could now trust other observations about how the plants used water and nutrients, and interacted with their carbon dioxide enriched environment. “With that kind of an approach we could measure the net gain in carbon or loss, and do it in correlation with temperature, rainfall, sunlight, you name it.”
As a demonstration that an experiment of that kind was possible, Drake never expected his project to become the foundation for a field site that would last three decades and inspire similar work in other environments around the world. It is now the longest running field study ever on the effects of rising carbon dioxide on a plant community, and it’s still going.
“While we’ve been studying it, the carbon dioxide in the atmosphere has come up something like 13 or 14 percent,” Drake says. “Sea level came up something like 10 or 15 cm (4 to 6 inches).” What’s more, he and the dozens of researchers who have now conducted experiments at the site have been able to observe the marsh through a full range of environmental conditions, from wet years to dry, from warmer years to cooler years, long growing seasons and short ones.
“Having such a long continuous study really gives us huge amounts of information that we simply can’t obtain any other way,” says Long. “[Drake] took on something completely new when he set it up. It was a very bold thing to do and it succeeded.”
One of Drake’s early findings was that increasing carbon dioxide to the marsh led to increased emissions of methane gas. They also learned that sedge plants didn’t overtake grasses, despite their ability to grow faster in a high carbon dioxide environment.
Each discovery led to more questions, and the field site grew exponentially. Scientists like Megonigal who followed Drake, have improved their design, switched out welded aluminum frames for PVC, enlarged the chambers and added more of them for additional studies. Along the way, new experiments have delved deeper into complex interactions in the ecosystem.
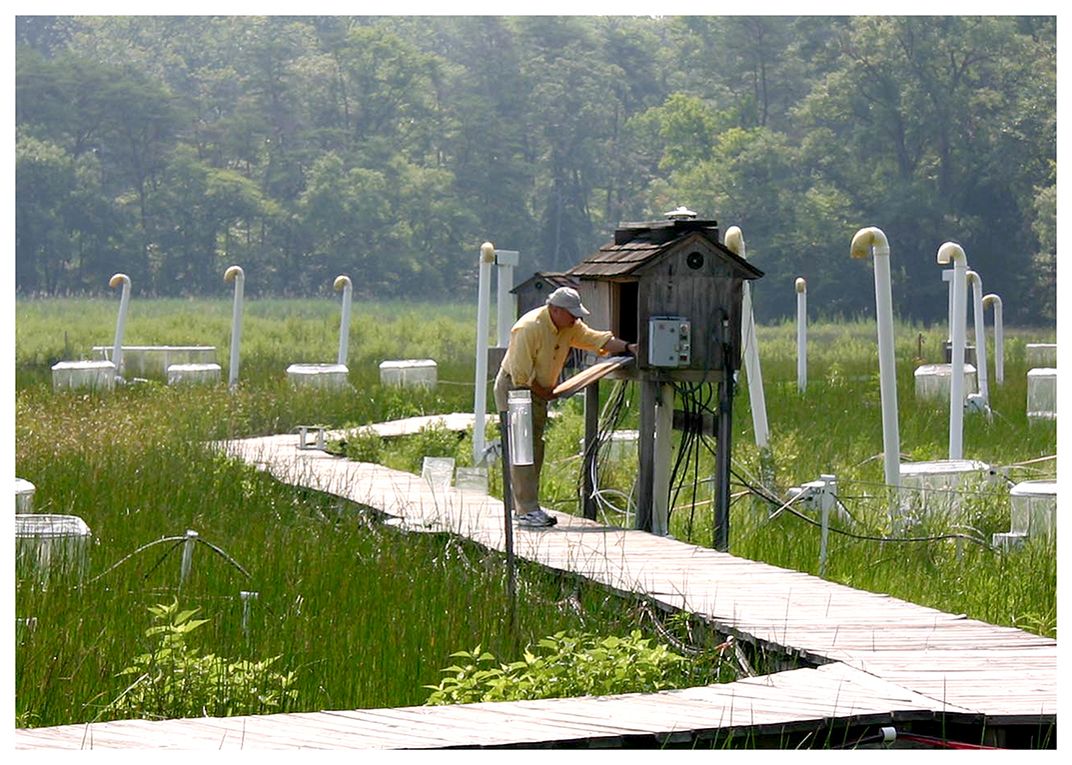
When the scientists increased nitrogen in the soil to simulate increasing runoff from land, they discovered that not all plants reacted the same, and their responses changed depending on the carbon dioxide and water available. One step at a time, they’ve been teasing apart important interactions, seeking a window on what the marsh may look like in the next 100 years.
In 2015, Megonigal published a study in which he and his colleagues subjected the plants to different water levels to see how they would respond to rising sea level. “We expected that as the marsh begins to submerge, it ought to be able to preserve more carbon and actually be able to keep up with sea level rise,” Megonigal says. Their thinking was that more frequent inundation with water would keep oxygen levels low in the top layer of the soil. That would slow the microbes that decompose dead plant roots and allow more soil to accumulate.
But that’s not what happened. Like little snorkels for microbes, the roots transport oxygen from the air down into the soil, which means it doesn’t really matter how long the soil spends under water. What matters is how many roots there are delivering oxygen to the microbes. Megonigal found that the more roots you have, the more decomposition occurs.
“The way decomposition is represented in models does not address the influence of plants,” says Megonigal. “So our models are, for the most part, are wrong, at least based on this one study. We need to focus on the combination of these things, because it’s their interactions that are going to be really important to understanding climate change.”
For policy makers, understanding the combination of factors influencing the survival of wetlands is about more than simply knowing what will happen. Active management of the land is going to be a crucial part of some nations’ strategies for keeping a lid on global warming.
According to Burkett of the U.S. Geological Survey, it couldn’t be more urgent. “[Wetlands] naturally emit methane but they also store billions of tons of carbon, and how they are managed influences the rates of carbon sequestration and release.”
Maintaining or restoring natural hydrology to wetlands may increase their ability to store carbon, whereas converting them to agriculture or shrimp ponds may release what’s stored in soil as carbon dioxide.
“A key message for policy makers is that wetlands are complex systems,” she says “To enhance the long-term storage of carbon in these wetland systems, you have to understand the biogeochemical cycling of carbon in them. That’s a scientific endeavor that will help support the commitment made in Paris by countries around the world.”
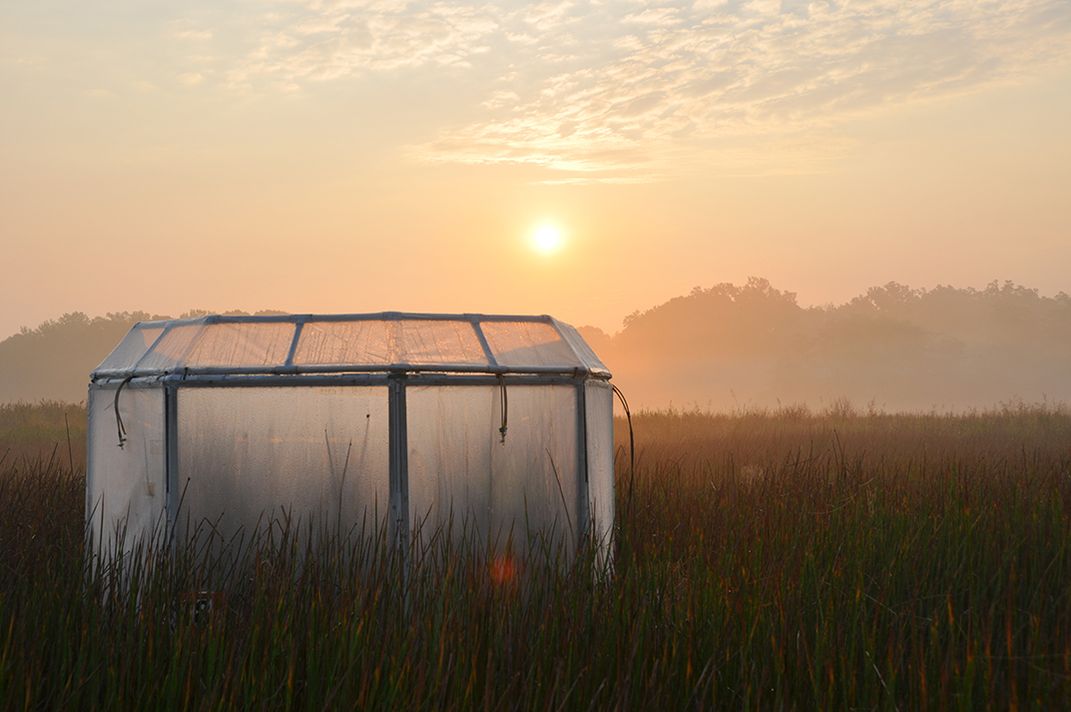