The First Sighting of a Black Hole
We know one lurks at the center of the Milky Way, but to these astronomers, seeing will be believing.
/https://tf-cmsv2-smithsonianmag-media.s3.amazonaws.com/filer/7f/5c/7f5c984a-2145-4fb6-a18a-f3baac8172b9/black_hole.jpg)
The center of the galaxy doesn’t look like much, even if you’re lucky enough to live in a place where the night sky is sufficiently dark to see the bands of the Milky Way. In visible light, the stars between here and there blur together into a single brilliant source, like a bright beam hiding the lighthouse behind it.
But in other types of radiation—radio waves, infrared, X-rays—astronomers have detected the presence of an object with the mass of four million suns packed into a region smaller than our solar system: a supermassive black hole.
Astronomers call it Sagittarius A*, or Sgr A* (pronounced “sadge A star”) for short, because it’s located (from our point of view) in the Sagittarius constellation. Discovering the Milky Way’s black hole has helped cement the idea that the center of nearly every large galaxy harbors a supermassive black hole. But despite mounting evidence for black holes, we still haven’t seen one directly.
That may change very soon. Astronomers are about to begin an observing project that may result in what was once thought impossible: a picture of the supermassive black hole at the center of our galaxy.
Many scientists—including Albert Einstein, whose 1915 Theory of General Relativity predicted black holes (though the term was coined more than 50 years later)—have doubted that nature could actually make such a monstrous thing: an object so gravitationally powerful that it would trap anything passing too close to it, including light. Then, in the 1950s, astronomers discovered quasars—objects so bright they were mistaken for nearby stars until their spectra proved they were billions of light-years away and receding fast. The immense energy released by quasars is too great to be explained by nuclear fusion, the engine that creates a star’s light.
“The discovery of quasars and radio galaxies told astronomers that not all the energy of the light we saw was from so-called thermal processes—heat,” says K.Y. “Fred” Lo, the director emeritus of the National Radio Astronomy Observatory, who died on December 16, 2016. Some astronomers began to speculate that perhaps this superabundance of energy was evidence of black holes. Perhaps as matter was falling into them, the immense pull of gravity was exciting the material and causing it to blast out “a phenomenal amount of energy,” says Lo.
Since the 1930s, astronomers have observed strong radio waves coming from the center of the Milky Way. After theorists suggested in 1971 that the source could be a black hole, several researchers decided to go black hole hunting in the region.
At the time, Lo was a graduate student at the Massachusetts Institute of Technology studying star formation regions—clouds of hot hydrogen plasma—which Sagittarius A contains. He looked at the region using a radio interferometer, a technique that was just being developed; interferometry uses multiple telescopes to create the observational power of a single telescope with a diameter equalling the distance between the networked telescopes. Lo used one radio observatory in Green Bank, West Virginia, and another just south of Washington, D.C., to get the resolution of a telescope 125 miles across. With this powerful observing capability, he spotted just a hint of a compact radio wave source—what astronomers believed would indicate a black hole. “As a student I was very cautious and I never published the result,” Lo says. “Nevertheless, it was there!”
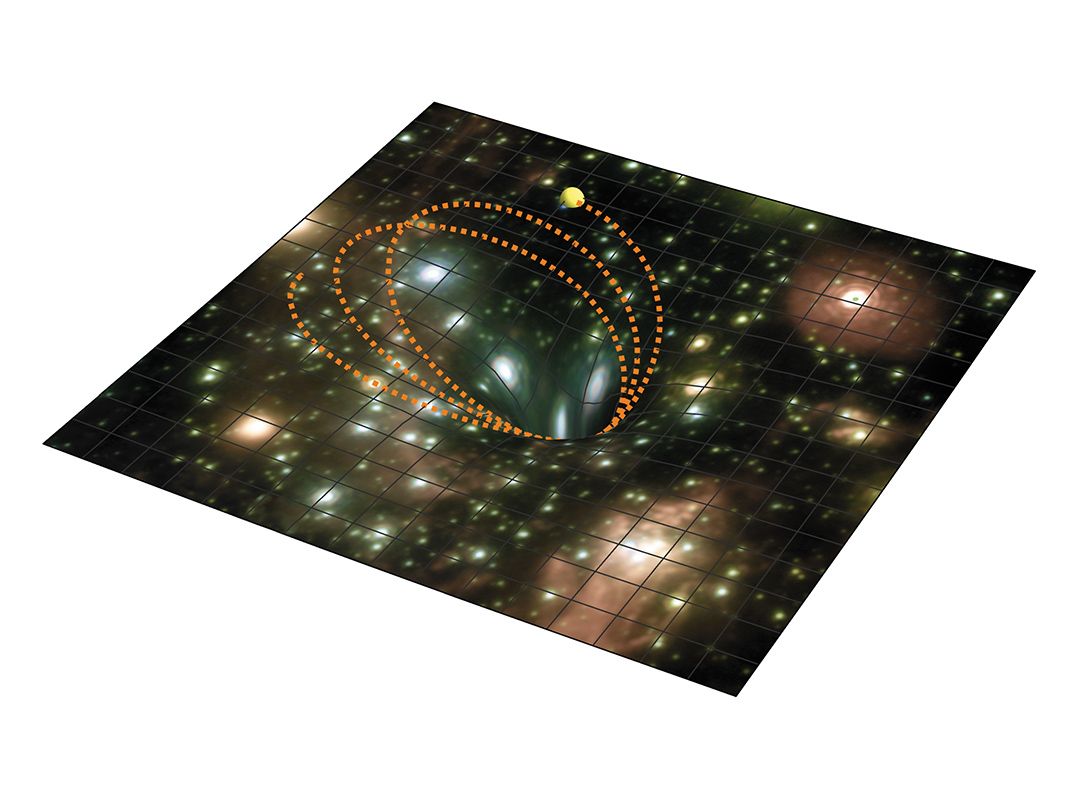
Lo was close enough to announcing that the Milky Way contained a black hole to spur two other astronomers, Bruce Balick and Robert Brown, into action. They used two radio telescopes in West Virginia to zoom in on Sagittarius A. Over two days in February 1974, Balick and Brown observed the galactic center at a high enough resolution to see a discrete, intense source of radio waves. Brown named the discovery Sagittarius A*—in atomic physics, the asterisk is used to refer to an atom in its “excited state,” and nothing was as exciting to Balick and Brown as discovering this black hole.
Two years later, Lo, using radio telescopes in California, confirmed Balick and Brown’s discovery, and he continued to study Sgr A* for the next 30 years. Astronomers quickly realized they would need to keep developing very long baseline interferometry to get the extremely high resolution required to make observations of Sgr A*. One result from astronomers’ increasingly detailed observations became a famous poster showing the center of the Milky Way in radio energy, which still hangs in many astronomers’ offices and classrooms.
While the first detection of Sgr A* was a major discovery, the observations didn’t reveal its mass or size, quantities necessary to determine whether an object is a black hole. In the early 1990s, German astronomers Reinhardt Genzel and Andreas Eckart used advances in infrared astronomy at the New Technology Telescope in Chile to look at the galactic center. The black hole is the brightest single-source radio wave emitter in the galaxy, but in infrared it is nearly invisible. Stars, however, show up clearly in infrared. Genzel and Eckart found 39 stars very close to Sgr A*, including several that appeared to be orbiting the black hole itself.
Around the same time, Andrea Ghez, now an astronomer at the University of California at Los Angeles, was a doctoral student looking at Sgr A* with the much larger Keck telescope in Hawaii. Both groups needed to overcome a major problem: Infrared observations are sensitive to atmospheric fluctuations, which cause stars to blur together. Ghez used a method called speckle imaging, which she credits as being the “breakthrough” in studying Sgr A*. “You take very short exposures, which freeze the interference effects of the Earth’s atmosphere,” she says. Those effects can then be removed using computer processing. The technique revealed that a few stars were orbiting Sgr A* fast enough that successive observations showed them changing position. Now the astronomers could calculate the speed at which the stars were moving, and extrapolate their orbits. Using the laws of orbital motion, they then calculated the mass of Sgr A*—four million times the mass of our sun—and placed a limit on its size: about the diameter of the solar system.
Today, both the German and the American groups have further improved their view of Sgr A* with adaptive optics, which compensates for atmospheric distortions by using a supercomputer to change the shape of a deformable mirror in real time. Astronomers have since been able to find several stars even closer to Sgr A*. “It’s given us the definitive proof for the existence of this supermassive black hole, and it’s given us an awesome ability to study the environment around the black hole,” says Ghez.
“The evidence is really quite compelling,” says Fred Lo. “By process of elimination, it can’t be anything else.” But, he adds: “Strictly speaking, no one has proved that there’s a black hole.” So now astronomers are looking for the final piece of the puzzle: an observation of the event horizon. This is the invisible barrier where the black hole’s escape velocity—the minimum speed at which an object must travel to escape the gravitational pull—is greater than the speed of light.
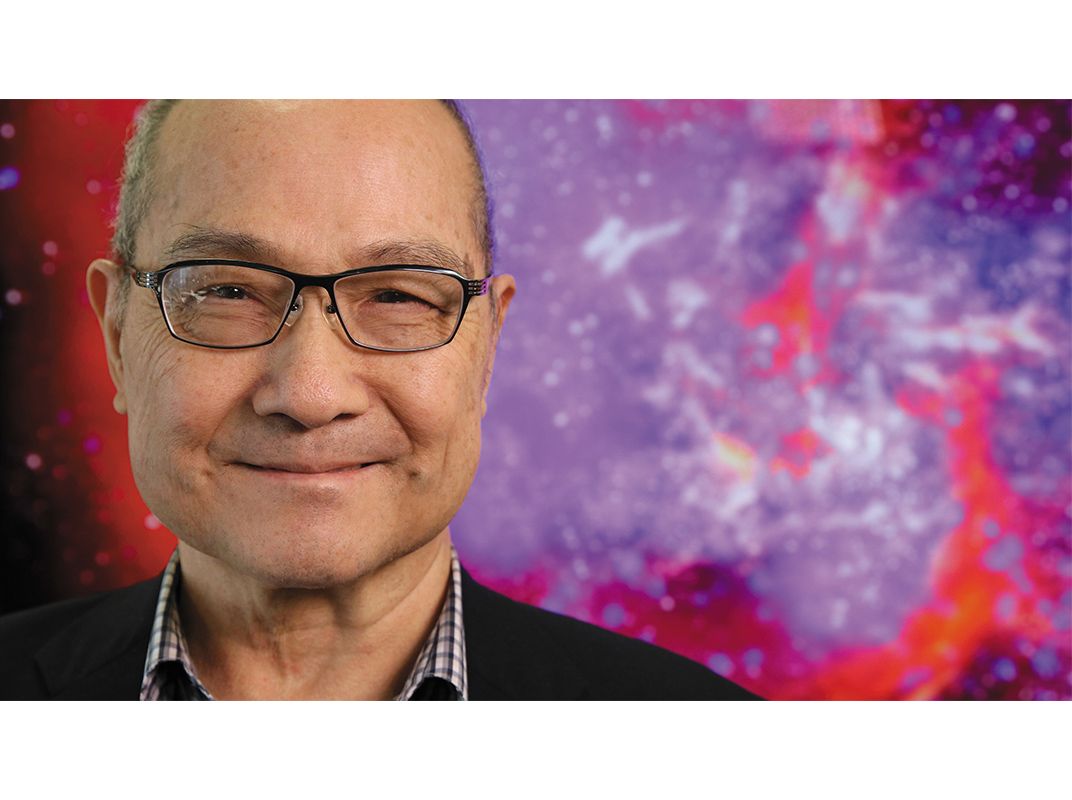
We can’t see the boundary itself, but there are ways to look for markers of the event horizon. Compared with stars or planets, black holes are remarkably simple: They are described completely by two numbers. The first property is mass—just the total, not its composition. Whatever fed it originally—types of atoms and particles, dark matter, planets or stars or old boots—is irrelevant.
The second property is spin. As a black hole spins, it drags matter and light into a whirlpool. The faster it spins, the more violent the whirlpool, and the more egg-shaped the event horizon becomes as it warps space-time around it. General relativity equations tell us that a star orbiting close enough to Sgr A* won’t trace a flat ellipse—the shape of an orbit under normal gravity—but will get tilted into three dimensions, creating more of a chrysanthemum pattern. The extravagance of the “petals” depends on the black hole’s spin rate. Ghez expects to collect enough observations to have evidence of that pattern within the next few years.
A real windfall would be the discovery of a pulsar in orbit around Sgr A*. Duncan Lorimer, an astronomer at West Virginia University, has been studying pulsars for 25 years but began to look closely at the galactic center only about five years ago. A pulsar is a very dense star that blasts out a streaming jet of radiation. As it spins, that beam crosses our view in regular intervals, which astronomers can time to precise degrees. A pulsar could indicate not only how the space around the black hole is warped, but also how the time is warped. “Einstein’s equations make very concrete predictions about how that clock [near a black hole] will be altered by the structure of space-time around it,” says Lorimer. “Even just finding one normal pulsar around the black hole will be phenomenally interesting.”
The stars that astronomers have found near Sgr A* have solved one mystery—the mass and approximate radius of the black hole—but they have also created others. Astronomers were surprised, for example, by the observation that the stars they’ve observed are quite young. “One would predict no young stars near black holes, because they have very strong tidal forces,” says Ghez. The gravitational pull should shred clouds of gas before they become dense enough to collapse into new stars. For a long time, astronomers believed that only old stars would be close to a black hole, because they would have been around long enough to migrate to the most massive thing nearby, says Ghez. This was so strongly believed that, until the math showed how dense the object at the galactic center was, many astronomers argued that the presence of so many young stars nearby was evidence that Sgr A* was not a black hole. Scientists call this “the paradox of youth,” and it’s one of the biggest questions about how supermassive black holes affect nearby star formation.
This is one reason astronomers got very excited in 2011 when they discovered that an enormous cloud of gas, which they named G2, was on course to make a very close approach of Sgr A*. Most astronomers assumed the black hole’s tidal forces would pull apart G2, releasing tremendous amounts of energy in bright flashes, like those observed when gas interacts with black holes in other galaxies. But in 2014, with all eyes eagerly turned to Sgr A*, G2 passed by without much fanfare, surviving nearly intact. When Ghez and her colleagues examined the data, they concluded that G2 couldn’t have been a normal gas cloud, but rather two stars hiding inside a single huge envelope of gas. Ghez thinks that the gravity of Sgr A* pulled the two stars into a tight orbit around each other and tugged their outer layers into a ragged mass 100 times larger than either star would have ordinarily. This might explain why there are more young stars around Sgr A* than expected. Instead of ripping apart potential stars, the black hole’s gravity is turning them into something new and weird.
Last summer, using a new suite of infrared interferometry instruments called Gravity, astronomers working with the European Southern Observatory observed a star that completes an orbit around SgrA* every 16 years. By combining data from the four telescopes that make up the Very Large Telescope in Chile, Gravity is able to precisely measure stellar orbits close to the event horizon. The ESO observers plan to compare the stars’ orbital movements with predictions made by Einstein’s theory. Gravity should also be able to track the occasional flare-up when Sgr A* consumes gas—which it does occasionally, despite its undramatic interaction with G2. Observing these outbursts is another way to calculate a black hole’s spin.
But the most ambitious project focused on the galactic center is the Event Horizon Telescope, an enormous interferometer made from a dozen observatories stretching from Hawaii to the South Pole. The goal, when it comes online this spring, is to capture an image of Sgr A* with enough resolution to see the event horizon itself.
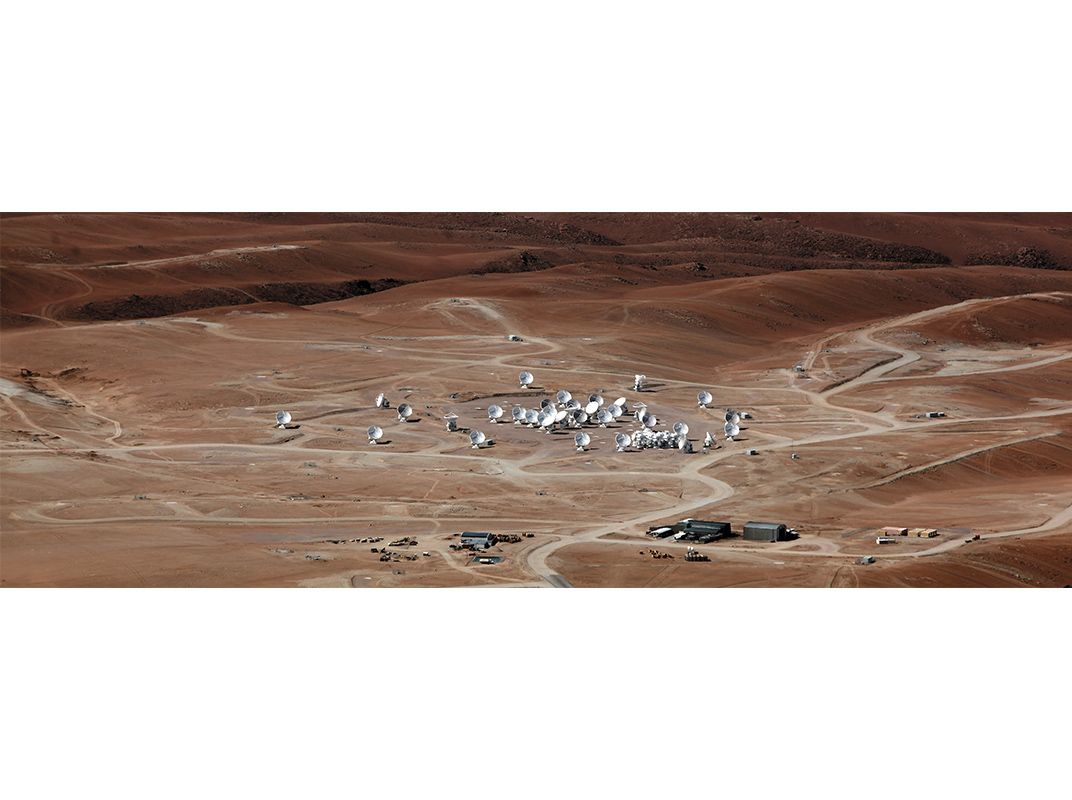
“Until the last handful of years, we didn’t actually think that we would be able to see the event horizon directly by taking a picture of it,” says astrophysicist Feryal Özel of the University of Arizona. “There are particular characteristics of our galactic center black hole that make it possible.” In 2000, Özel and her colleagues showed that matter falling into Sgr A* doesn’t itself obscure the horizon. The first observations with the entire array will take place in early April, and involve roughly a week of continuous monitoring of Sgr A*. If all goes as planned, humankind will get its first real look at one of the most bizarre objects in the universe.
What will it look like? “We should see a silhouette, a shadow,” Özel says. Sgr A* will show up as “an absence of light that is roughly circular imprinted on the surrounding radiation.” (The film Interstellar includes a decent visualization of a black hole shadow, based on calculations from general relativity.)
For people studying black holes and gravity, measuring the shape and size of this shadow will be a pinnacle achievement. “Proving that event horizons exist will be extremely exciting,” says Özel. “It’s not just the existence of the black hole,” but how this information will provide an unprecedented way to study the warping of space-time that the general relativity theory describes. The event horizon will need to be a very specific size, or all the assumptions about Einstein’s theory and what Sgr A* really is will come into question. “It’s a no-wiggle-room test.”
As astronomers have slowly revealed the true nature of Sgr A*, “what we’re seeing is nothing like what we expected,” says Andrea Ghez. And a black hole portrait may be the biggest surprise of all. Whatever the result, our curiosity will continue to pull us toward the powerful, mysterious object at the center of our galaxy.