The Telescope That Will Change Astronomy
After years of delay and frustration, the James Webb Space Telescope is ready to launch.
:focal(577x585:578x586)/https://tf-cmsv2-smithsonianmag-media.s3.amazonaws.com/filer/b7/92/b792ce04-1842-4405-a782-7ea0531591b3/07a_on2021_jwsttourbatterypark_live.jpg)
Sometime this fall, if the launch date doesn’t slip again, NASA’s James Webb Space Telescope—the most powerful, expensive, and eagerly anticipated telescope ever to gaze out into the universe—will lift off from French Guiana on an Ariane 5 rocket. Twenty-six years in design, delayed countless times, the JWST, as astronomers typically refer to it, will dwarf the Hubble Space Telescope in size, resolution, and ability to see very faint objects. Unlike Hubble, which operates primarily in the visible spectrum, Webb will use cameras and spectrographs tuned to near- and mid-infrared wavelengths to view some of the oldest objects in the cosmos, with a sensitivity 1,000 times better than past infrared space telescopes. Webb’s shiny gold-covered mirrors will collect photons that have been journeying our way since a few hundred million years after the Big Bang.
Is the JWST remarkable? Unarguably. Is it needed? Space scientists would say yes. Webb will uncover mysteries about our cosmic origins that are beyond the capability of the 31-year-old Hubble or any other observatory, in space or on the ground. The new telescope is completely novel in design. To block warm sunlight from hitting its super-cold infrared detectors, Webb has a sunshield as big as a tennis court, composed of five layers of Kapton film, each no thicker than a plastic baggy. Along with other types of armor, the shield also will help protect against meteorite impacts. The telescope’s gigantic primary mirror, 6.5 meters in diameter (Hubble’s was just 2.4 meters), is a mosaic of 18 tightly fitting hexagonal segments, and the whole thing has to be folded up like the leaves of a dining room table to fit inside the Ariane rocket nose cone for launch.
During a journey of nearly a month to reach its home in space, the telescope will deploy and unfold its many parts in a heart-stoppingly complex sequence. Unpacking and extending solar arrays, antennas, booms, radiators, mirrors, and the sunshield itself (which deploys like a layered parasol), the telescope will cool down to temperatures around minus 400 Fahrenheit, all the while kept facing away from the sun, moon and Earth to maintain its infrared sensitivity.
Its final destination is a stable orbit around Lagrange point 2, or L2, a million miles from Earth—an equilibrium point that balances the gravitational forces of the sun, Earth, and moon so that the telescope needs minimal fuel for orbit corrections. Unlike Hubble, the JWST is not designed to be upgraded. Hubble was saved from ignominy in 1993 by an astronaut repair crew correcting for the misshapen mirror the telescope had launched with three years earlier. There will be no such rescue if something goes terribly wrong with Webb; the observatory has to perform flawlessly. Making sure it will has cost NASA a great deal of time, money, and stress as engineers test, retest, and try to build in enough redundancy—“two of everything”—to ensure against failure.
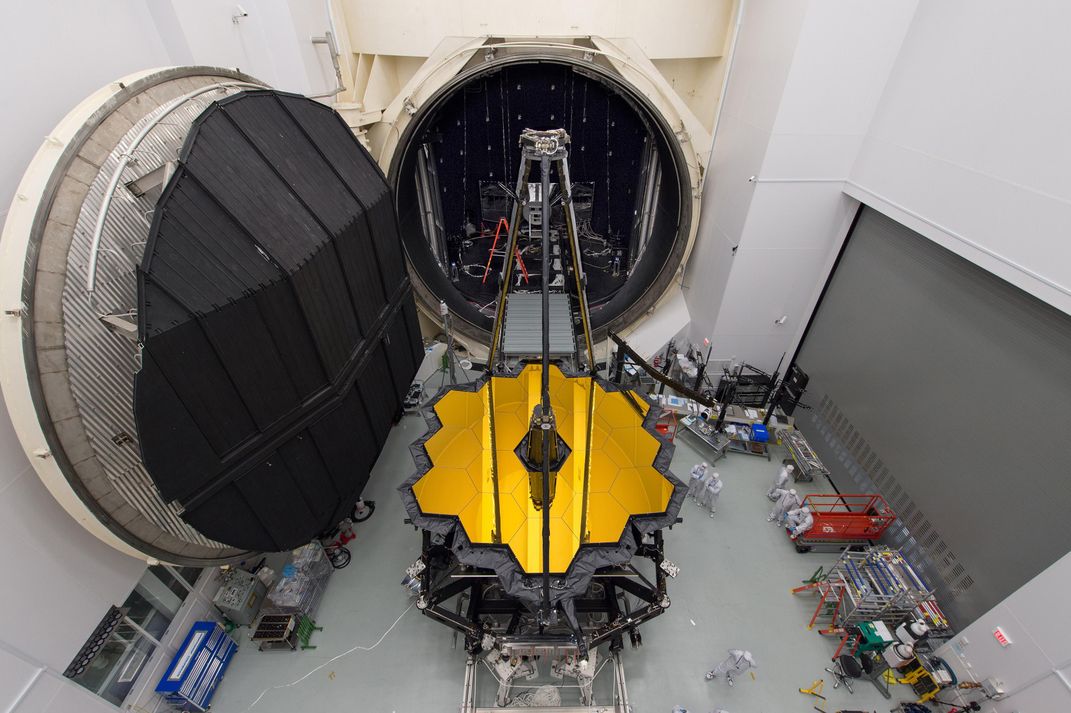
“This is the most complex observatory and spacecraft ever attempted,” said Klaus Pontoppidan, an astrochemist and project scientist for JWST science operations at the Space Telescope Science Institute in Baltimore. Just as it does for Hubble, the institute acts as the telescope’s nerve center, producing software for ground control systems, coordinating the observatory’s initial deployment, scheduling science observations for astronomers worldwide, and receiving and archiving all of Webb’s data. “I have confidence in the people doing the deployments and hardware,” he said, “and I think it’s going to work well.”
John Mather, a senior astrophysicist and Webb project scientist at NASA’s nearby Goddard Space Flight Center, started working on the project—then called the Next Generation Space Telescope—in 1995. (The name was later changed to honor the administrator who guided NASA through the Apollo moon landings in the 1960s. After a petition accusing Webb of involvement in a 1950s purge of LGBTQ government employees circulated last May, the name might change again.) When he started working on Webb, Mather was just winding down from his key role on the Cosmic Background Explorer (COBE) project, which precisely measured the universe’s primordial glow of microwave radiation and earned Mather a Nobel Prize in physics in 2006.
“COBE was done, and I got a phone call from NASA about this next-generation telescope,” he recalled. “ ‘Did I want to be a head scientist? Of course!’ But I didn’t understand it would take forever.”
As Mather tells the story, Alan Dressler, a senior astronomer at Carnegie Observatories who studies galactic evolution, helped convince then-NASA administrator Dan Goldin that a very large infrared telescope was needed in space, one that could reach back in time about 13 billion years to detect the earliest stars and galaxies. Scientists believe that during this epoch, a few hundred million years after the Big Bang, clouds of neutral gas condensed to form the first luminous objects. Due to the expansion of the universe, light from these early stars and galaxies has been shifted from its original shorter wavelengths into the infrared. Unfortunately, Hubble can’t see far enough into that region of the spectrum. NASA’s Spitzer Space Telescope (2003-2020) could, but, with a mirror less than a meter in diameter, it didn’t have the ability to see very distant objects.
“With Hubble, especially, we had hoped to be able to see galaxies being born, but we can’t,” Mather explained. “These objects are too far and too faint. Their light is more red-shifted than Hubble can receive. So [JWST] will help us understand how the first smooth universe [developed] seeds that would grow into objects, galaxies, and black holes.” Mather hopes that JWST’s acuity will also “tell us about gravitational waves in this early material. If gravitational waves were there—or not—makes a big difference in our understanding of basic physics.”
/https://tf-cmsv2-smithsonianmag-media.s3.amazonaws.com/filer/8d/51/8d514035-3d52-410f-a2c1-00bfa9294bcf/07b_on2021_jwstsunshield_deployment_live.jpg)
Budget and Technical Woes
When Mather signed on to the Next Generation Space Telescope, he invited fellow Goddard astrophysicist Eric Smith, who had experience in spaceborne astronomy, to collaborate on the telescope’s science requirements and early design. The two set to work with technical committees, engineering teams, and aerospace contractors to create concepts and drawings for the new space observatory.
Right from the start, the scope of the project was underestimated, and its execution was woefully underbudgeted. The original estimates in 1996 for a smaller telescope came in at $1 billion to $3.5 billion, with a projected launch date as early as 2007. Today’s price tag exceeds $9.3 billion.
The overruns didn’t come as a total surprise. “Dan Goldin was a great enthusiast for getting things done cheaper,” Mather recalled. “The response in the first meetings was laughter: ‘Ok boss, we’ll try.’ But you couldn’t do [that telescope] for the budget he asked for.”
Smith, who is now the program scientist for Webb at NASA headquarters (he has held that position for Hubble as well), said the structure and size of the telescope kept morphing along with the complexity of ground equipment and testing systems needed to ensure flawless operation in space. For example, new processor chips had to be developed to meet very challenging low-noise performance requirements. Non-magnetic beryllium was chosen as the mirror substrate—instead of the silica glass used in Hubble—thanks to its light weight, stiffness, strong electrical conductivity, and ability to hold its shape across a range of extreme temperatures. To increase their reflectance, the mirrors had to be coated with a gold layer only 120 nanometers thick, applied through vapor vacuum deposition.
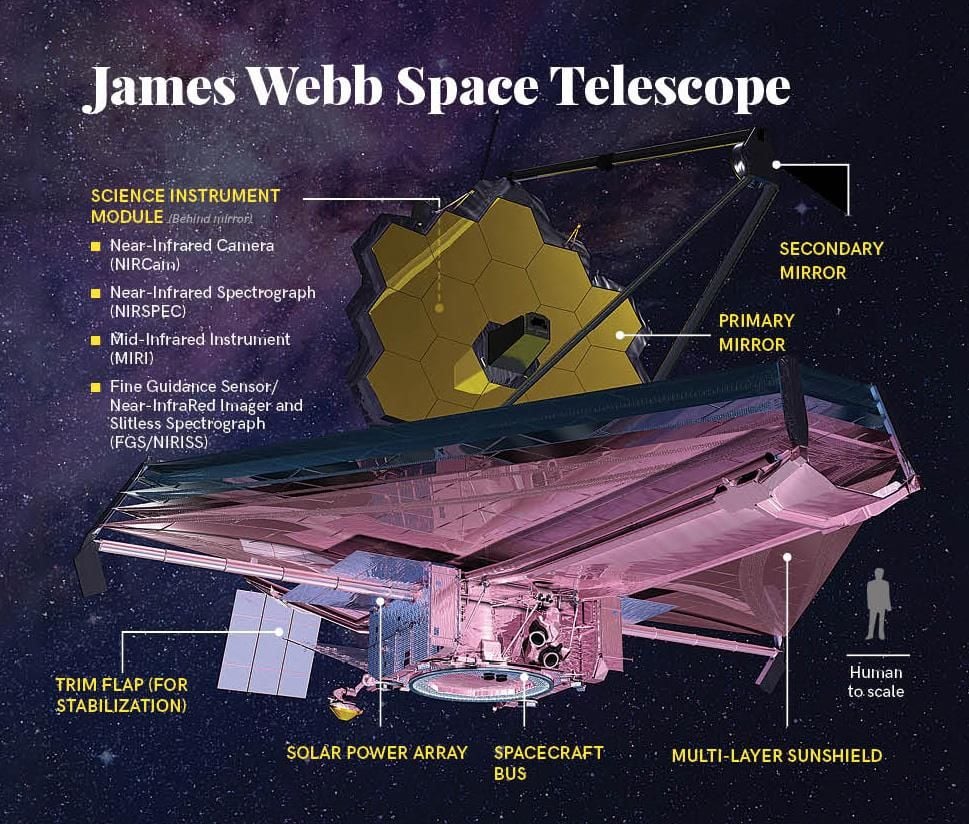
Such innovation costs money. “Many astronomy missions have relied on military and space agency counterparts [for their technical heritage],” Smith said. “But we [NASA] are paying the full freight for most of this,” although Europe and Canada, as partners, have contributed money, staff, and science instruments, along with the Ariane rocket launch.
The project’s political and budgetary problems mounted. Even after main construction of the telescope began in 2008 and it passed its critical design review in 2010 with excellent technical scores, cost overruns prompted Congress to demand an independent review. A year later, a House of Representatives appropriations committee even tried to cancel the project by reducing NASA’s overall budget by $1.9 billion. But by November 2011, the critics backed down, in part because JWST received strong endorsement from the American Astronomical Society and influential Senator Barbara Mikulski went to bat for an expensive project based in her home state of Maryland. Congress then capped the telescope’s budget at $8 billion. That total has been far exceeded.
Smith offers an explanation. “We never built anything like this before,” he said. “The [initial] project was under-scoped, and later on we had a recognition of how technically difficult this was going to be.” For one thing, the telescope mirror that was needed to get the required sensitivity would exceed the size of any rocket fairing, the topmost part that encapsulates the spacecraft. So the mirror had to be segmented and folded inside the nose cone.
“We also knew we needed a deployable telescope,” said Smith, one that would unfold in a complex sequence of operations and take up its post a million miles away instead of in Earth orbit like Hubble. And because of the desire to detect very faint infrared radiation coming from the farthest reaches of the universe, the observatory itself has to emit as little thermal energy as possible. “We’d have to cool it and keep it cool, and that leads to a sunshield and a tube that blocks out [stray] light,” said Smith. “But the tube has mass, and you want to minimize the amount of mass you’re launching. [So] we knew that if we’d have to put this telescope far from Earth, which is a big infrared light source (along with the sun and the observatory’s own electronics), you’d need a parasol—a one-sided shield facing the sun, Earth, and moon. The mirrors live on the cold side.”
NASA needed new ground equipment to test all the complicated unfolding. And trying to mimic the zero-G dynamics of this one-of-a-kind spacecraft in normal gravity was a big job in itself, especially when it came to testing the five-layered sunshield, which has to be carefully “tensioned” and tightened in space after it unfolds. In 2018, much to NASA’s embarrassment, the sunshield cover shed some of its screws and washers during vibration and acoustic tests. That prompted another delay while an independent review board looked into that and other technical issues.
Hopes and Fears
At its peak, as many as 2,000 scientists and technicians were working on Webb, with a total of about 10,000 people involved in its construction over the decades. Northrop Grumman is the telescope’s prime contractor, having bought TRW, the original contract winner, in 2002. Apart from the spacecraft, mirrors (primary and secondary), and sunshade, four instruments had to be designed and built to analyze light collected by the mirror. Spectroscopy is just as important as imaging, as it breaks the incoming light into discrete wavelengths that can reveal the source object’s chemical makeup, motion, and other physical properties.
The University of Arizona is providing the Near-Infrared camera, or NIRCam. The European Space Agency (ESA), in cooperation with the Jet Propulsion Laboratory (JPL), supplied the Mid-Infrared Instrument (MIRI), a combination camera and spectrograph that sees even farther into the infrared.
ESA also built NIRSpec, short for Near-Infrared Spectrometer, which is especially suited to studying distant galaxies and can analyze light from more than 100 sources at a time. “It’s the first time this has ever been done in space,” said Nora Lützgendorf, a NIRSpec instrument scientist. That unique capability comes from a novel microshutter array whose cells (averaging the width of a human hair) have tiny lids that open and close individually when a magnetic field is applied, enabling the instrument to selectively view or block targets in its field of view.
A final instrument, called FGS-NIRISS, short for Fine Guidance Sensor/Near Infrared Imager and Slitless Spectrograph, built by the Canadian Space Agency, is a “big cosmos” spectrograph that can measure the spectral colors of all the celestial objects in its field of view at the same time. The FGS part of the instrument helps Webb lock onto targets with great precision. “It’s probably the best guidance sensor ever built,” said René Doyon, a professor of physics at the Université de Montréal and principal NIRISS investigator.
“NIRISS and NIRSpec are complementary,” explained Doyon. “Whereas NIRSpec can select individual objects…for NIRISS there are no slits, and hence we can take spectra for a few thousand sources in the field but at the expense of a lower sensitivity.” NIRISS is optimized to measure the spectra of relatively bright stars along with massive galaxy clusters and low-mass galaxies whose light is distorted (a phenomenon known as gravitational lensing) by other massive objects in the line of sight between them and the telescope.
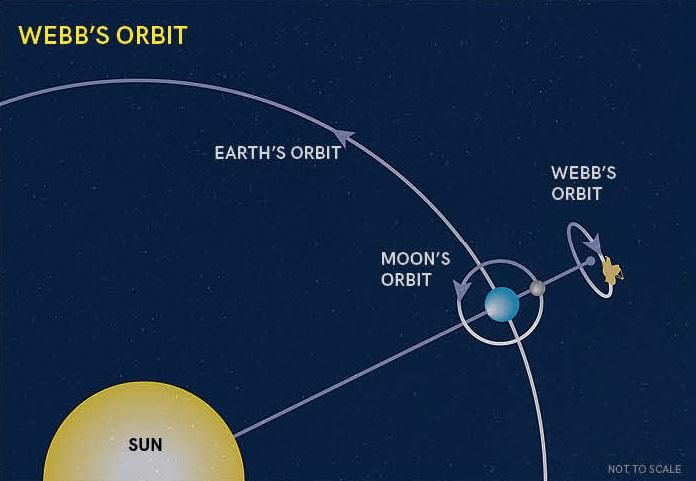
As launch day approaches, astronomers worldwide are as nervous as they are excited. Thomas Zurbuchen, who heads NASA’s science office, channeled their collective anxiety when he wrote recently that the rocket ride itself won’t even be the worst part: “About 50 deployments need to occur after launch to set up the huge system. With 344 so-called single-point failures—individual steps that have to work for the mission to be a success—this deployment after launch will keep us on edge for three weeks or so. For comparison, this exceeds single-point failures for landing on Mars by a factor of three, and that landing lasted only seven minutes….Those who are not worried or even terrified about this are not understanding what we are trying to do.”
The first six months after launch are devoted to commissioning the observatory—making sure the spacecraft and instruments work as designed. Another five months or so will be dedicated to early release science observations. Select astronomers will train the telescope on a wide range of targets, from distant galaxies to planets in our own solar system, to gain experience with the nuances of this new instrument and share that knowledge with the rest of the astronomical community—somewhat like Air Force test pilots wringing out a new fighter jet before it gets deployed to flight squadrons.
Other early users will be the so-called “guaranteed time observers,” people who have spent years of their lives planning and building this new telescope and so will get first crack at using it, without having to compete for observing time. The JWST was designed primarily for deep-space cosmology, but any telescope of this caliber does a little bit of everything, and it will be called on to do virtually every kind of astronomical observation—whenever and wherever its unique sensitivity and power is required. (See below for a sampling of these guaranteed time studies.)
Kuiper Belt
/https://tf-cmsv2-smithsonianmag-media.s3.amazonaws.com/filer/d7/4d/d74d4c73-59d0-45b8-aaeb-d47476bf491f/180_webb_space_telescope_expert.png)
Jonathan Lunine, Cornell University
So wide-ranging are Jonathan Lunine’s scientific interests—he’s studied everything from Mars to protoplanetary disks—he had no trouble thinking of ways to use his allotted time as a JWST interdisciplinary scientist. One of his projects will look at small bodies at the edge of our solar system—specifically, the Kuiper Belt objects Quaoar and Haumea and several asteroid-comet hybrids called Centaurs, which have migrated in from the outer reaches.
These are thought to be leftovers from the solar system’s formation, and Lunine is most interested in compositional differences, not just among these primordial objects, but within them. Knowing what kinds of ices like methane and carbon monoxide they contain, and in what proportion, helps scientists understand their evolution. Webb’s mid-infrared instrument will take spectral data for different longitudes on Haumea’s surface as it rotates.
Even with Webb’s resolution, the pictures won’t show any real detail on such small, distant bodies. “People shouldn’t expect images,” says Lunine, “but they should expect to find out what these things are made of.”
—Tony Reichhardt
Exoplanets
/https://tf-cmsv2-smithsonianmag-media.s3.amazonaws.com/filer/91/07/91070e74-c54b-4f7d-b729-240f038c0ebc/180_webb_space_telescope_expert2.png)
Nikole Lewis, Cornell University
In 2018, when she was still with the Space Telescope Science Institute, Nikole Lewis co-led a team that used Hubble to study the TRAPPIST-1 planetary system, 40 light years away.
Now Lewis is back with an even more powerful telescope to look at TRAPPIST-1e, one of three rocky worlds in this nearby system that might be habitable. Hubble showed that none of the three has an extensive hydrogen atmosphere like Neptune. That’s good. It means that TRAPPIST-1e might be more like Earth, which it roughly matches in size. The Webb telescope can look deeper, for other gases in the atmosphere that might indicate the presence of life.
The first goal is to verify that the planet even has an atmosphere, which, says Lewis, is “still not clear.” Then she’ll “be looking at relative abundances of things like carbon dioxide, water, and methane in the atmosphere to see if their ratios hint at a habitable planet.”
Before moving to Cornell, Lewis was the JWST project scientist. Given the recent explosion of interest in exoplanets, she thinks it’s a happy development that a telescope originally designed for cosmology turns out to be so useful for studying other solar systems. Twenty-five percent of Webb’s observing time will be devoted to exoplanets.
—Tony Reichhardt
Black Holes
/https://tf-cmsv2-smithsonianmag-media.s3.amazonaws.com/filer/78/92/789286e5-bcd3-4f49-9fb7-a2a08afeaf34/180_webb_space_telescope_expert3.png)
Roeland van der Marel, Space Telescope Science Institute
The center of the Milky Way galaxy can be considered the middle distance for JWST’s vision—only 26,000 light years away, compared to billions of light years away. Astronomers have long known that a good-size black hole sits at the center, gobbling up all the mass in its vicinity.
The trouble is, dust obscures all but a few bright stars near this danger zone. Webb’s sharp infrared vision can pierce through the dust and see much fainter stars, adding to the number of objects that help astronomers understand the dynamics of such an extreme environment. Images taken over a number of years can detect the motion of stars caught in the whirlpool, and the telescope might also see stretched-out clouds of gas, the remnants of stars torn apart by gravitation.
Roeland van der Marel is already working on NASA’s next big space observatory after Webb, called the Nancy Grace Roman Space Telescope,
which is due to launch in the mid-2020s. First, though, he’ll use some of his team’s guaranteed time to study the universe at its most violent.
—Tony Reichhardt
Early Galaxies
/https://tf-cmsv2-smithsonianmag-media.s3.amazonaws.com/filer/e6/d3/e6d3ca63-3f4d-4526-b944-caa9f9a64cb5/180_webb_space_telescope_expert4.png)
Marcia Rieke, University of Arizona
Marcia Rieke’s family may be unique in all the universe. She’s principal investigator for Webb’s NIRCam instrument. Her husband George, also an astronomer at the University of Arizona, is the U.S. science team leader for MIRI. Between them, they’re responsible for half the instrument suite on the world’s premier telescope.
The study of early galaxies, JWST’s primary job, will involve dozens of scientists. A whopping 800 hours of observing time is reserved for the JWST Advanced Deep Extragalactic Survey (JADES), co-led by Marcia Rieke. Webb will take long exposures of a patch of sky known as GOODS-S, (for South), which has already been intensively studied by astronomy’s most powerful telescopes, across the spectrum from radio to X-rays.
What might Webb see that the others haven’t? “The shapes [of these first galaxies] are one of the big questions,” says Rieke. “If you go by what the theorists think is going on, we should see a collection of relatively small, irregular shapes. And then as we look at lower and lower redshifts [progressively later in time] we’ll see the effect of those little blobs having joined together under the influence of gravity to form more and more regular shapes.” It will be like watching the baby universe grow up in time-lapse.
How long will it take scientists to piece together the data? Says Rieke: “I’m hoping that in three to six months we might be able to give an interesting press conference with at least preliminary results.”
—Tony Reichhardt
Finally, astronomers around the world can apply for precious time using Webb, in annual cycles wherein their proposals are peer-reviewed and the winners awarded observing time. For “Cycle 1,” as the first projects are called, 6,000 hours’ worth of these community observations were approved, to study everything from active galactic nuclei and supermassive black holes to stellar populations, planetary discs, exoplanets, and the large-scale structure of the universe.
A project called COSMOS-WEBB is the largest of these general observer programs selected for the telescope’s first year. JWST will focus on the celestial equator, said Jeyhan Kartaltepe, an astronomer at the Rochester Institute of Technology, who co-leads a team of 50 scientists. “We’ll create a physical map of the earliest galaxies with the highest redshifts,” she said. “With Hubble, we could study galaxies…out to z~6 [a measure of redshift] and in not a lot of detail. With JWST, we can go all the way out to z~10 with improved resolution at all redshifts.”
Caitlin Casey, the COSMOS-WEBB co-investigator and an astronomer at the University of Texas in Austin, said she expects to see galaxies dating from only 300 to 400 million years after the Big Bang: “That’s a small fraction of the age of the universe and well beyond what Hubble could do. The whole premise…is that we’re not just going to find galaxies, but [discover]…how localized or clustered they are.”
For John Mather, the Nobel winner who has been working on this project since before Kartaltepe and Casey were in high school, launch day has been a long time coming. “For me, I’m thrilled to see that an idea written in a little book by Dressler’s committee has now become real,” he says. “The power is astonishing. I feel privileged that people can actually make this flight hardware work.”
As for what JWST might tell us about the universe, “I’m hoping to find something that no one has truly imagined,” says Mather. “Nature has some tricks…and I think we’re going to see them.”