Drones’ Newest Cargo Might Just Be Human Organs
Surgeon Joseph Scalea is developing a cooler, biosensors and an online platform with GPS to monitor organs in transport in real time
/https://tf-cmsv2-smithsonianmag-media.s3.amazonaws.com/filer/35/41/3541f40d-61dc-485a-81df-f40d8530f916/organdeliverybydrone.jpg)
Unmanned aerial vehicles, or UAVs, are frequently used for tasks considered too dangerous for traditional aerial surveillance—mapping ice floes in the Arctic, for example, or monitoring forest fires in California. Because they are relatively cheap, small, portable and maneuverable under cloud cover, drones have been deployed extensively in geographical surveys, environmental disasters, surveillance and image recording. Over the past few years, however, improved ability to detect patterns, obtain data in real-time, and detect obstacles make these flying robots ideal for conveying some extraordinarily precious cargo: human organs.
Joseph Scalea, assistant professor of surgery at the University of Maryland Medical Center, has begun testing drones outfitted with coolers and biosensors that can monitor an organ’s health throughout its aerial journey—the first design of its kind in the last 65 years of organ transport. Scalea has applied for a patent for his technology, “Human Organ Monitoring Apparatus for Long-distance Travel” (HOMAL), which measures the biophyisiologic properties (temperature, pressure, vibration, altitude) of an organ. This device, together with an online platform that features organ GPS, allows physicians and hospitals to view the location and status of the organ in real time, almost like a pizza delivery or Uber car service. While transplant science is an arguably evolving field, Scalea’s project takes research bench to bedside, thereby increasing the viability of blood and tissue samples that need to be rapidly shepherded over distances of hundreds of thousands of miles.
Before UAV transport of organs can become a clinical reality, however, some significant hurdles remain. What ethical objections, if any, will donors, patients or their doctors have to the idea of sending an organ on an unpiloted drone? Will the organ deteriorate during flight? How will hospitals and the aviation industry accommodate an influx of unmanned flying robots in the country’s limited airspace? Finally, who will be held responsible if a drone fails to deliver its organ to the intended recipient on time, or at all?
When a patient needs an organ, every second matters. In surgery, this critical period is known as cold ischemia: the time between the chilling of an organ after its blood supply has been reduced and the time it is warmed by restoring its blood supply. From the moment it’s removed from the body, tissue begins to deteriorate, making speedy transport a priority. But the current system of getting a kidney or a heart from point A to point B involves a complex network of couriers and commercial aircraft—meaning frequent delays, missed connections, even lost organs.
Some 33,000 deceased organs are transplanted and transported every year in the U.S. Once they are removed from donors, livers, hearts, eyes, spleens and other body parts are carefully packaged and preserved on ice (a process that takes up to two hours), before they begin their journey with a series of couriers. First, organs must be transported to the airport, where they await a commercial flight (this can take up to 10 hours), then to baggage handlers, who load organs with other freight; often, a second chartered flight (a helicopter) takes the organs to the destination hospital, where they are unloaded by handlers and held for blood retrieval and biopsy, before they are moved again by courier to an organ blood bank, where a surgeon can at last retrieve them.
The entire process typically takes 24 hours (and that doesn’t account for delays on the tarmac) and costs an average of $6,000, while a chartered flight—the more common mode of transport for organs needing to fly between hospitals in different cities—can exceed $40,000. Scalea’s technology promises dramatic travel time and cost savings: given a total travel distance of 1,000 miles, for example, and a drone flying at 200 miles per hour (half the speed of commercial aircraft), an organ could be moved from hospital A to hospital B in five hours, with two hours on each end for packaging and transplant, thereby eliminating more than 50 percent of travel time. The current system, with its numerous connections and opportunities for delay, thus makes drone-delivery of organs a viable alternative, particularly in instances where an organ recipient is thousands of miles from his or her donor.
Scalea contends daily with the challenges of organ transport, an undertaking where the stakes are often life-or-death. “As a surgeon, I love being able to tell people that they’re going to get 10 more years to live,” he explains. “To learn that I can’t do that because an organ missed its connecting flight, for example, is beyond common sense.” Scalea was determined to develop an alternative. He knew that the technology already existed; the real challenge was to cultivate strategic relationships—with engineers, manufacturers, investors, clinicians and private airline carriers—in order to overcome the formidable logistics of getting a body part from one point on the globe to another. “Organ transport is my passion and my mission,” says the surgeon. “Re-innovating it has become my career goal.”
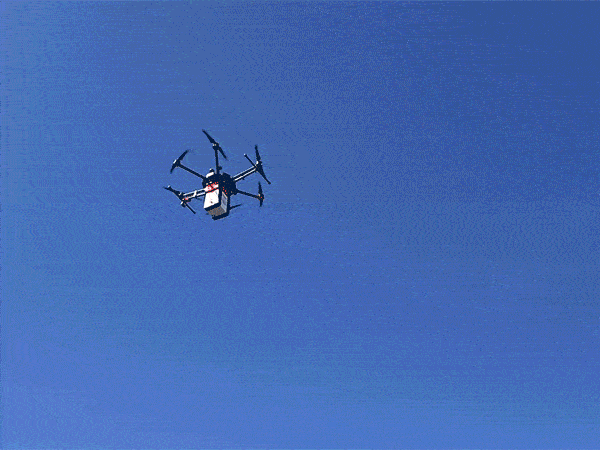
Three years ago, Scalea reached out to the University of Maryland’s Department of Engineering and set to work building a prototype, along with technology that would let both a physician and a drone’s controller monitor the status of an organ along its airborne route. The team selected DJI M600 Pro for their experiment because its six motors lie directly below their respective rotors, meaning rotors are kept far from a smart cooler compartment. That separation would ensure that an organ would be spared from any heat generated by the drone’s motors. Real organs were used during the three-mile test flight in March of 2018 and carefully monitored from takeoff to landing; they displayed no physiological problems after their aerial journey.
The team faced a couple initial challenges— to make a drone small enough such that it would not add significant weight to the payload, and to assess whether changes in altitude would affect organ viability. (It turns out that organs, just like scuba divers, can suffer “the bends” when they ascend to altitude too quickly.) In addition to static testing on the ground—making sure communication between the app, the IT platform and the device is secure—Scalea also assessed his prototype in various temperatures and vibrational forces. Future tests will try to predict organ function in changing environments.
At the same time, Scalea worked to develop his private company, Transplant Logistics and Informatics, and established a formal partnership with the United Network for Organ Sharing, the nonprofit organization that manages the nation's organ transplant system.
He also began a dialogue with the Federal Aviation Administration (FAA), the governing body that may ultimately decide the fate of drone-assisted organ delivery. Currently, aviation law restricts drone flight to less than 400 feet above the ground, at a speed of 100 miles an hour or less, and mandates that drones are flown in line of sight—that is, with a visible path between the UAV and controllers.
The law won’t necessarily need to change in the immediate future, since the FAA currently administers specified waivers for drones, but a more specific set of regulations may be needed if drone-delivering organs become the norm. Although the drone used in Scalea’s experiment flew out just a mile and a half and back, the team is looking to phase in longer distances (the average organ flight between hospitals in the U.S. is about 400 miles) and designing its models accordingly. The next step? Performing an actual transplant using drone delivery—a feat that may be possible in less than a decade, according to Scalea.
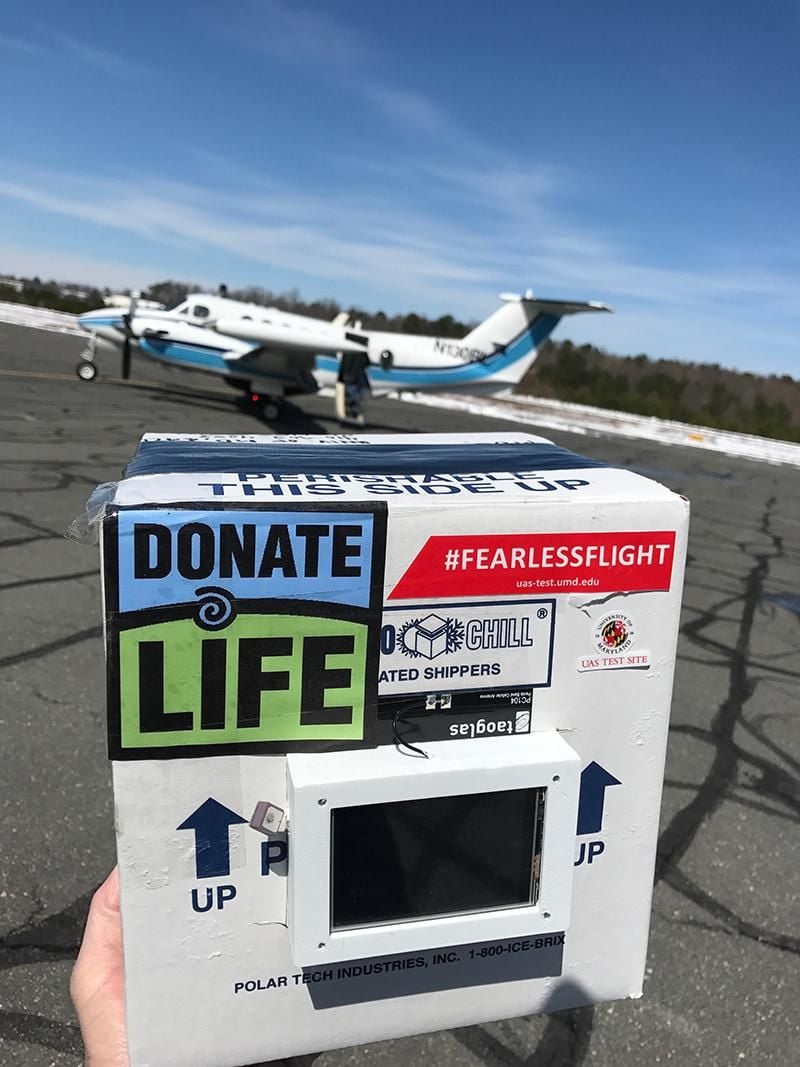
As UAVs become an urban traffic reality, one key (and not-at-all-trivial) challenge is to keep drones from running into other objects: planes in the air, pedestrians on the ground, birds or buildings somewhere in between. From an engineering perspective, that means a clear design of both the machine and its mission. A drone used for kidney delivery between two hospitals in the same city might look very different from one used to transport blood from Columbus to Cleveland, for example; weight and power requirements will differ depending on payload, distance and flight speed, which all must be defined at the outset.
Wind and visibility pose added complications for drones, which cannot currently fly through ice or cloud-cover—mechanical problems that are formidable but not insurmountable, according to Jim Gregory, professor of mechanical engineering at Ohio State University and director of the University’s Aerospace Research Center. Gregory specializes in the intersection of aerodynamics and drones, an area of research that includes everything from drone path planning in a gusting wind environment to ground control situational awareness.
When flight testing UAVs, Gregory (who also enjoys piloting planes in his spare time) emphasizes three crucial factors: the ability to detect and avoid obstacles, to maintain a robust control link between drone and ground-based operator, and the ability to verify a machine’s autonomy—that is, proving the safety of an autonomous system. “There’s a good case to be made for drone delivery of organs,” he says. “What makes it easier than, say, Amazon’s air package delivery idea, is that an organ-delivering drone will travel from one well-controlled environment to another well-controlled environment,” he explains. Indeed, hospitals are already equipped with helipads that can receive organ-bearing UAVs, and much of the infrastructure for delivery is already in place.
Gregory’s latest project involves a 33-mile stretch of air that travels through airspace in Columbus, Ohio. “We’ve created a kind of corridor for safe UAV traffic,” he says. This highway in the sky, funded by the Ohio Department of Transportation, might soon serve as a designated path for drones; the hope is that more like it can be developed in conjunction with city planners.
To that end, ground controllers will stay informed throughout the drone’s journey, in what might someday make up a system of “unmanned aircraft-traffic control.” At present, most drones report their position by onboard GPS—similar to the systems used by air-traffic control for commercial airplanes. But when humans travel 35,000 feet above the Earth, the FAA also monitors our craft via radar: a transponder repeatedly broadcasts its location through something called Automatic Dependent Surveillance Broadcast (ADS-B). Of course, FAA surveillance of drones is a new frontier, and one that will no doubt be debated in earnest at the FAA conference in Baltimore this June. “I don’t know that the FAA has defined exactly how drone surveillance will work,” says Gregory. “Some advocate for ADS-B, but the system could become overloaded if there are so many drones flying around.”
In the short-term, Scalea’s organ-delivering UAVs can reduce cold ischemia times and improve survival rate for isolated patients awaiting organ transplant; long-term, they may help us maximize organ allocation—that is, eliminate the geographic constraints presently put on organs, so that they can go anywhere at any time—essential to the expansion of organ donor pools throughout the world.
"The future is more imminent than we all think," says Scalea.