Five Scientific Findings That Could Lead to New Inventions
From cat tongues to dandelions seeds, engineers often look in peculiar places for inspiration
/https://tf-cmsv2-smithsonianmag-media.s3.amazonaws.com/filer/c2/6a/c26ad25d-eb36-4de2-900e-9d7824e09872/dandelion.jpg)
British architect Michael Pawlyn thinks of nature as a “catalog of products,” all of which, he explains in a TED Talk, “have benefited from a 3.8-billion-year research and development period.”
“Given that level of investment,” he goes on to say, “it makes sense to use it.”
While new technology can sometimes feel strange, almost other-worldly at first, the future of innovation actually involves researchers better understanding the natural world around us. And inventors are catching on, with more and more embracing biomimicry, or the process of designing products to function as animals and plants do after evolution’s fine-tuning. From mantis shrimp to bee spit, engineers are leaving no stone unturned when it comes to inspiration.
Here are five recent discoveries in the natural world that might someday lead to new inventions.
Mantis shrimp have a super tough armor made of an impact-resistant microstructure.
Mantis shrimp are feisty little devils that don’t back down from a fight—even with their own kind. Remarkably, two mantis shrimp can duke it out and remain unscathed afterward. That’s because the tough tiny fighters are covered in super-strong armor down their backs. The armor, called telsons, look and act sort of like shields, overlapping as they cascade down the crustacean’s tail.
Researchers at the University of California, Riverside studied the structure and mechanics of these telsons and found that the key to their toughness seems to be the spiral-shaped scaffolding underneath each shield. In a recent study in the journal Advanced Functional Materials, the engineers and their colleagues explain that the helicoidal structure prevents cracks from growing and softens the impact of a tough hit. A similarly twisted architecture is known to exist in the shrimp’s claw, used to deliver blows to any threats to its territory. The shrimp have clearly evolved the perfect armor.
Someday, we might see this sort of impact-resistant microstructure, which the researchers patented in 2016, in sporting equipment, body armor for the police and military, drones, wind turbine blades, aerospace materials, cars, military vehicles, airplanes, helicopters, bicycles and marine vessels. Basically, David Kisailus, a professor of chemical and environmental engineering at University of California, Riverside, and study author, explains in an email to Smithsonian magazine, “Anywhere reduced weight is critical but toughness and strength are required.”
Kisailus thinks, in the near term, the finding will have the biggest impact on sporting goods, because the time to market for products like helmets and shin guards is shorter than it is with something like commercial airplanes. The researchers have made a prototype helmet for construction use as well as for football. But, Kisailus adds, “in the longer term, I think the bigger, more global impact will be in transportation, since the reduced weight with higher strength will reduce fuel consumption and emissions.”
Dandelion seeds reveal newly discovered form of natural flight.
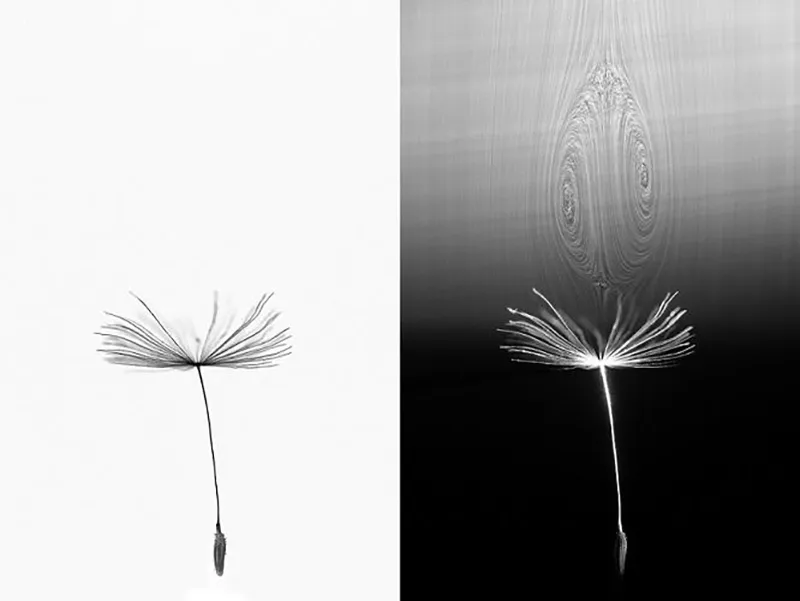
The way dandelion seeds effortlessly drift in the wind, catching glistening sunlight as they fall to the ground, has a certain simplistic beauty to it that would be hard to top. But, as researchers found last fall, the invisible path its delicate bristled parachute leaves behind is even more wondrous—and studying it could lead to really cool advancements in drone flight and air pollution monitoring.
Researchers knew that the mechanism that carried the seeds so effortlessly was its delicate crown of ivory fibers, which sort of resemble a chimney sweep’s broom. They just weren’t sure exactly how this parachute-like fuzz worked given that the bundle of dandelion seeds is mostly made up of empty space. So scientists at the University of Edinburgh created a wind tunnel to put the seeds to the test and in doing so, they discovered a “new class of fluid behavior,” reports James Gorman for the New York Times. The air flows through the filaments and leaves a swirling trail of air behind, or what’s called a separated vortex ring. The ring increases a seed’s drag, creating a flight four times more efficient than that of a conventional parachute.
The researchers, who explained the finding in a study published in Nature, hope it inspires engineers to invent tiny self-propelling drones that would require little to no energy consumption to fly.
“A dandelion-inspired man-made bundle of bristles could be used to float around in the air, carrying something like cameras or sensors, instead of the seeds,” says Naomi Nakayama, a biologist at the University of Edinburgh and study author, in an email to Smithsonian. “Just as the dandelion, they could stay afloat for a long time, being able to monitor and record the air quality, wind direction or speed, and perhaps some human activities, without people noticing they are around because they are so tiny.”
Mako sharks are speedy because of their flexible scales.
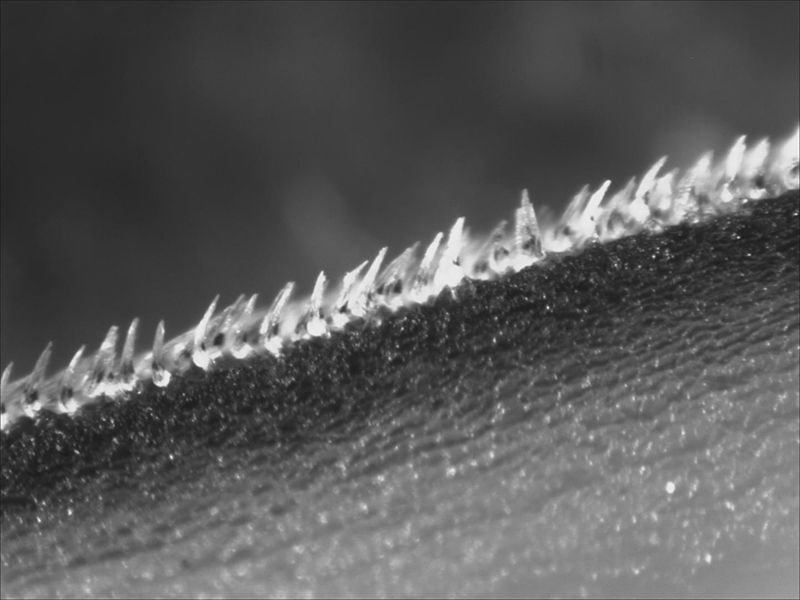
Mako sharks are freaky fast, which is why they’re sometimes called the cheetahs of the sea. They can reach up to 70 to 80 miles per hour. But how do they get so fast? The answer lies with tiny scales on their flank and fins. But exactly how their slick skin helps their speed is of special interest to aeronautical engineers, with funding from Boeing and the U.S. Army, who want to design new material to reduce drag and increase the agility of aircraft, according to an American Physical Society press release.
The flexible scales on mako sharks’ flank and fins are just one-fifth of a millimeter long. If you were to pet the shark like a cat, from head to tail (Editor’s Note: We don’t advise this.), its scales would feel smooth. But if you ran your hand in the opposite direction, the skin would feel more like sandpaper, with the scales bending backward to a maximum 50-degree angle depending on body location, with the most flexible scales behind the gills. According to the press release, the scales’ flexibility keeps the flow moving forward close to the skin, preventing what’s called “flow separation.”
Flow separation is also enemy number one when it comes to aircraft. The concept is easily demonstrated by sticking your hand out of a moving car window with your palm facing the wind. Your palm is under more pressure than the back of your hand, and so your hand gets pushed backwards. This happens because the air flow separates around the sides of your hand creating the low-pressure region or wake behind your hand. Flow separation can still happen on a more streamlined body like the shark, however. That’s where the scales come in: they help control flow, thereby reducing the drag and letting the animal swim faster and with greater maneuverability.
“We speculate that at some point we could engineer a tape that could be applied strategically on aircraft surfaces, such as helicopter rotor blades, wings or certain locations on the fuselage where flow separation is occurring and causing an increase in drag or decrease in performance or maneuverability,” says Amy Lang, an aeronautical engineer at the University of Alabama, who presented the work at the American Physical Society March Meeting in Boston, in an email to Smithsonian.
Lang received a patent in 2014 that she says was “based on early concepts we had about how the shark skin functions and how we could apply that to an engineered surface.” She and her team are making 3D printed models of mako shark skin and hope to obtain more results from testing them in wind and water tunnels within the next year. “We hope in our collaborations with industry to file an updated patent as a man-made surface is developed for real applications,” she adds.
Bees combine spit and flower oil to make an adhesive.
Honeybees fly from flower to flower collecting pollen and storing it on their body to take back to the hive. But what if a surprise summer rain shower interferes? Never fear, bees have a solution for that: a sticky slurry of their spit and oils from flowers that turns pollen into water-resistant pellets. The science behind this gooey combination may even inspire high-tech glues that stick when you want them to but also release when necessary.
“We wanted to know, if the pollen can stay so firmly attached to the bee’s hind legs, how do the bees manage to remove it when they return to the hive,” said Carson Meredith, an engineer at Georgia Tech and lead author on a study published in Nature Communications in March, in a press release.
It essentially works like this: Bee spit is a little sticky to begin with because of the nectar they drink. The spit covers pollen when the bees collect it. Then the oils from the flowers coat the spitty pollen ball. This layering technique is the perfect concoction to repel unanticipated humidity.
“It works similarly to a layer of cooking oil covering a pool of syrup,” Meredith said in the release. “The oil separates the syrup from the air and slows down drying considerably.”
Speed is also a key factor it seems. That comes down to what’s called a rate-sensitive response, meaning “the faster the force attempting to remove it, the more it would resist,” according to a press release. So when the bees use coordinated, slow movements with their hind legs to remove the pollen balls, they come off easily. But if a free-falling raindrop collides with one of the balls, it adheres more intensely.
The applications for an adhesive like this vary widely. Meredith explains in an email to Smithsonian magazine that a bioinspired adhesive would thrive in areas where strength isn’t the top priority, but “where adhesion needs to be tailorable, tunable, responsive to stimuli, or coupled with other properties like edibility, biocompatibility or humidity resistance.”
He is working with both medical and cosmetic companies. (If you’ve ever found yourself removing stubborn waterproof make-up, you understand the demand for a solution.) “In these fields one often wants adhesion that can hold surfaces together under certain circumstances, but then can be released on demand or when a certain condition (rate, force, humidity) is exceeded,” he explains. “This includes the ability to transfer small particles from one place to another, as in applying make-up, or delivering a drug to a certain tissue in the body.”
That’s not all: those pollen pellets are naturally edible, so it could be used in food as well, perhaps for “decorative items on a cake or dessert, or adhering particulates that contain food additives for taste, nutrients, preservatives, color, etc.,” explains Meredith.
Cats are expert groomers because of hollow papillae on their tongues.
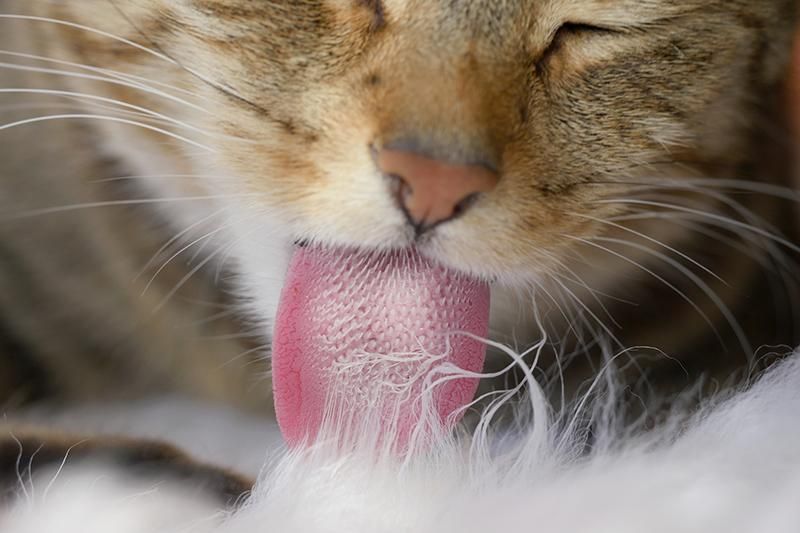
Cats spend a pretty significant amount of their time licking themselves. It turns out their tongue has evolved for peak grooming efficiency—and might actually help us make better hairbrushes, or even inspire advances in soft robotics and new kinds of cleaning tech.
A cat’s classically sandpaper-y tongue is covered in angled spikes called papillae, which are made of keratin, or the same hard stuff of our fingernails. That’s the part of the tongue that researchers at the Georgia Institute of Technology were interested in studying to find out exactly how it distributes moisture throughout a cat’s fur so easily.
It turns out papillae aren’t actually spiky, or cone-shaped as past research posited. Rather, as the Georgia Institute of Technology engineers describe in a study in the Proceedings of the National Academy of Sciences, they’re scoop-shaped with two hollow ends. This shape creates surface tension that locks saliva droplets in until it’s time for a cleaning, the team found. And those tongues can hold a lot of fluid. When the team put cat tongues—donated post-mortem—to the test, they found that each papilla can hold about 4.1 microliters of water, but across the tongue that’s enough to distribute about a fifth of a cup of water through the animal’s fur in a day, according to National Geographic.
The papillae also attack a knot from four different directions—perfect for efficient detangling. The researchers even created a tongue-inspired grooming (TIGR) brush using 3D models of cat tongues. They’ve applied for a patent for the brush, which could be used to apply medication or distribute leave-in shampoos and conditioners in pet fur to reduce allergens.
And the team envisions other applications. “The unique spine shape could be implemented into soft robotics to aid in grip—previous studies have shown that micro-hooks excel in gripping to porous, rigid surfaces,” says Alexis Noel, a research engineer at the Georgia Tech Research Institute and study author, in an email. There may even be a novel way to apply mascara, she adds.