This Lab-Grown Mini Heart Can Keep a Beat
The creation, called a cardioid, will help with the study of heart disease and the discovery of new medications
/https://tf-cmsv2-smithsonianmag-media.s3.amazonaws.com/filer/8c/26/8c260acd-f99c-4f99-a648-b2bbbfe81452/beatingcardioid1.gif)
A lab in Vienna has used stem cells to create thousands of tiny heart-like structures. Each miniature heart, dubbed a “cardioid,” is the size of a sesame-seed and has a hollow chamber that beats.
Cardioids differ from past heartlike inventions, which scientists created by using outside scaffolds and cells that they moved around—like constructing a wall out of bricks. Those creations aren’t as useful for studying realistic heart structure and disease.
The cardioids described today in the journal Cell develop without the training wheel-like guidance of an outside scaffold. The scientists instead introduced the stem cells to a series of chemicals that play important roles in heart development. The cardioids grow from bundles of stem cells into millimeter-wide water balloons in just one week. Most of the cardioids are a lot like the heart’s largest chamber, the left ventricle.
“You see the cells changing shape [while they grow] and it's really incredible, but it's on another level when they actually do something,” says Nora Papai, a biologist at the Austrian Academy of Sciences’ Institute of Molecular Biology and co-author of the study. “They start twitching at day five or so, and at day seven you see this nice pace of beating.”
In the future, these lab-grown mini-versions of human hearts could be used to study heart development and heart disease—replacing current methods that use the hearts of lab animals.
A cardioid is a type of organoid, a miniature version of an organ grown in the lab for use in research. There are some that resemble miniature brains, miniature guts and almost every other major organ. But no self-organizing heart organoids—developed from stem cells and chemical signals alone—had been created until now.
Unlike hearts, cardioids aren’t connected to a circulatory system, so they don’t have any piping running in or out of their cavity. And unlike hearts, which have four chambers, cardioids only have the one. But their similarities to hearts go beyond the cardioids’ little heartbeat. Cardioids have the same three layers as natural hearts, and their chamber is filled with liquid.
Cardioids can also handle environments that would stress out most test tube-bound clumps of cells. The researchers figured this out because of a shipping mishap: when they sent a batch of cardioids to a colleague who wanted to use them to study the pandemic coronavirus, the package got stuck in an airport. The cardioids spent four days at room temperature, swimming in a nutrient-filled liquid, before reaching their destination.
“They were still beating,” says Institute of Molecular Biology researcher Sasha Mendjan, lead author of the study, of the cardioids. The inadvertent stress test showed how robust the cardioids are. “Once they formed, they are happy. The process of formation, of course, it's more delicate.”
The cardioids begin as pluripotent stem cells, which have the potential to turn into any cell in the body given the right instructions from the environment. The research team used six chemicals known to be important in human heart development in their experiment. Other attempts at creating heart-like organoids used only two or three factors, and often ended up with non-heart cells in the final product. Mendjan suspects that some stem cells go rogue and develop into other cells because they aren’t getting a full set of instructions. Using all six factors, Mendjan says, was key to the cardioids’ success because it gave the researchers control over many pathways of molecular development.
When the team first designed their experiments, they were trying to develop stem cells into heart cells in a two-dimensional sheet, but the cells wouldn’t stay flat as they grew. The cells kept buckling and not sticking to the bottom of the dish, even with a layer of glue-like material on top.
So co-author Stefan Jahnel suggested letting the cells grow in a three-dimensional space instead. Then the cells had the space to take their balloon-like shape.
Once they had a reliable way to create cardioids, the researchers began to use them to test the heart’s response to different injuries. They created some cardioids with genetic mutations, and found that they resulted in smaller cardioids with proportionally less space committed to the chamber. This is the beginning of studying heart defects in early human development, which affect around two percent of children.
“There's no way for us to look at this in the human embryo at that stage because women don't even know that they're pregnant by that stage,” says Mendjan. The cardioid mimics the appearance of an embryonic heart after about the first month of development. So cardioids may be able to help with the study of defects like hypoplastic left heart syndrome, which appears early in development and is deadly without an invasive surgery.
The team also tested how the cardioids recover from simulated heart attacks.
“During a heart attack, almost one billion cells die, but they don't just disappear,” says Papai. To mimic the effect of many dead cells left behind on the cardioids, Papai and colleagues used a thin metal rod, about the size of the pin used to remove SIM cards from smartphones, that they dipped in liquid nitrogen to reach minus-320 degrees Fahrenheit.
“When you hold it into the cells, that area immediately dies off due to the very cold temperature. But when the cells die, they don't disappear,” says Papai. The cells stay behind on the cardioid, which then starts to recover.
Some cardioids that were designed to resemble embryonic hearts were able to regenerate themselves with living cells. But the cardioids that were more like mature hearts, with an added outer layer, instead developed a layer of collagen, like scar tissue. Scar tissue doesn’t pump or move as well as healthy heart tissue, so over time it can cause problems for a person who has had a heart attack. But because scientists have found that some cardioids regenerate, they want to start studying the detailed mechanisms of how, and maybe find a way to create better treatment after heart attacks.
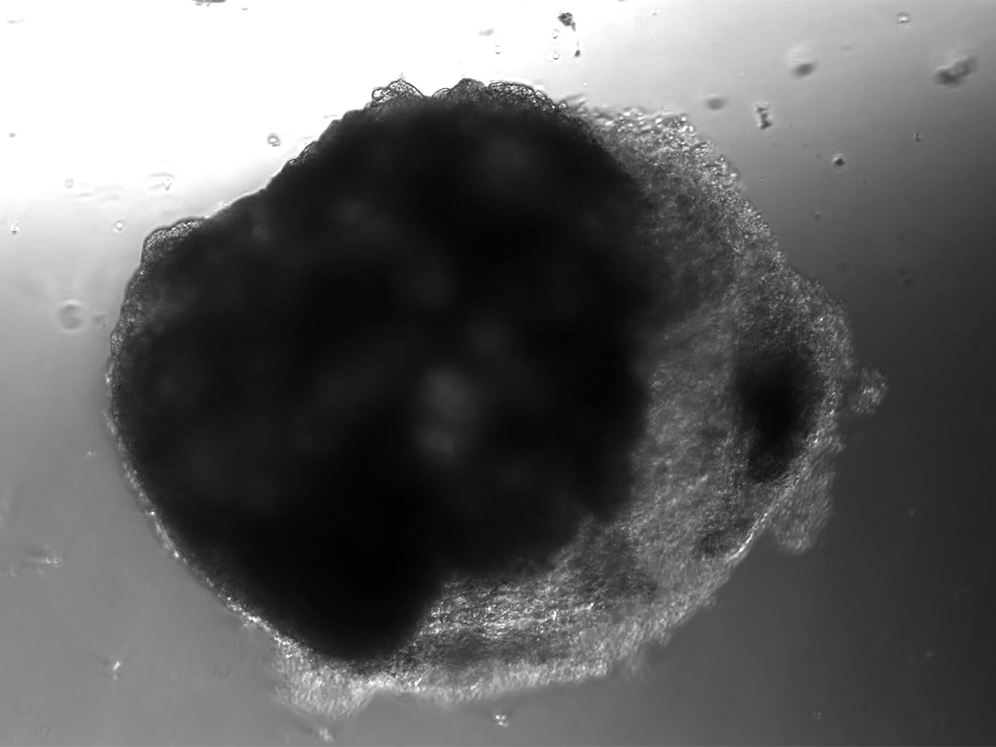
“Remarkably, Mendjan and colleagues overcome a major hurdle in the field to generate self-organizing human cardioids…by harnessing the normal rules of heart development,” writes Massachusetts Institute of Technology biological engineer Laurie Boyer, who studies the genes involved in heart development and was not involved in the new research, in an email.
The study brings the field “one step closer” to creating lab models for studying organ development and disease, says Boyer, but key advancements remain to be seen, like having models with all of the blood vessels, chambers and nerves of a real heart.
The research team is now patenting their cardioids and hope that the creations will provide a useful way to study heart development and test new drugs for heart diseases. The team has already found that cardioids can respond to the chemistry of their environment much like hearts do.
“This is now a bit creepy, but the amazing thing is that you can stimulate them to beat faster,” says Mendjan. “If you do these tests and you add, for example, adrenaline to them, all of them just wake up and then start beating very fast. And this is the same drug you would use to get a patient out of a heart attack.”