Let Us Now Praise the Invention of the Microscope
Early scientists wielded this revolutionary tool to study the invisible world of microbes, and even their own semen
/https://tf-cmsv2-smithsonianmag-media.s3.amazonaws.com/filer/7f/95/7f95e627-3288-4be4-a8d9-7ecf56b292be/microscope_image3.jpg)
Brad Amos has spent most of his life thinking about and looking into tiny worlds. Now 71 years old, he works as a visiting professor at University of Strathclyde in Scotland where he leads a team of researchers designing an extremely large new microscope lens—about the length and width of a human arm. Named one of Physics World’s Top Ten Breakthroughs of 2016, the so-called Mesolens is so powerful that it can image entire tumors or mouse embryos in one field of view while simultaneously imaging the insides of cells.
“It has the large coverage of a photographic camera lens and the fine resolution of the microscope objective, so it has the advantages of the two approaches,” says Amos. “The images are extremely useful.”
Today, microscopists like Amos are working around the world to innovate new technologies with widespread applications in medicine and human health. But these cutting-edge advancements all trace back to the very first microscopes built in the 16th and 17th centuries. While cutting-edge for the time, they wouldn’t impress you much; that weren’t much stronger than a handheld magnifying glass.
Amos has been obsessed with even these simplest of microscopes ever since he got one for a birthday as a kid. His intrigue in microscopic worlds became insatiable as he explored anything he could find, from the force within tiny, popping bubbles to the way pieces of copper molded under the poke of a needle. “It’s like play dough, it can be very soft,” Amos says of copper. He describes his awe at the phenomena he discovered under the scope that he couldn’t see with his bare eyes: “You are studying a world which doesn’t even obey the same rules of perception.”
This type of curiosity in the going-ons of tiny worlds propelled microscopy from its inception. A Dutch father-son team named Hans and Zacharias Janssen invented the first so-called compound microscope in the late 16th century when they discovered that, if they put a lens at the top and bottom of a tube and looked through it, objects on the other end became magnified. The device laid critical groundwork for future breakthroughs, but only magnified by between 3x and 9x.
The quality of the image was mediocre at best, says Steven Ruzin, a microscopist and curator of the Golub Microscope Collection at the University of California at Berkeley. “I have imaged through them and they are really quite awful,” says Ruzin. “The hand lenses were much better.”
Although they provided magnification, these first compound microscopes couldn’t increase resolution, so magnified images appeared blurry and obscured. As a result, no significant scientific breakthroughs came from them for about 100 years, says Ruzin.
But by the late 1600s, improvements to the lenses increased the quality of the image and the magnifying power to up to 270x, paving the way for major discoveries. In 1667, English natural scientist Robert Hooke famously published his book Micrographia with intricate drawings of hundreds of specimens he observed, including distinct sections within the branch of a herbaceous plant. He called the sections cells because they reminded him of cells in a monastery—and thus became the father of cellular biology.
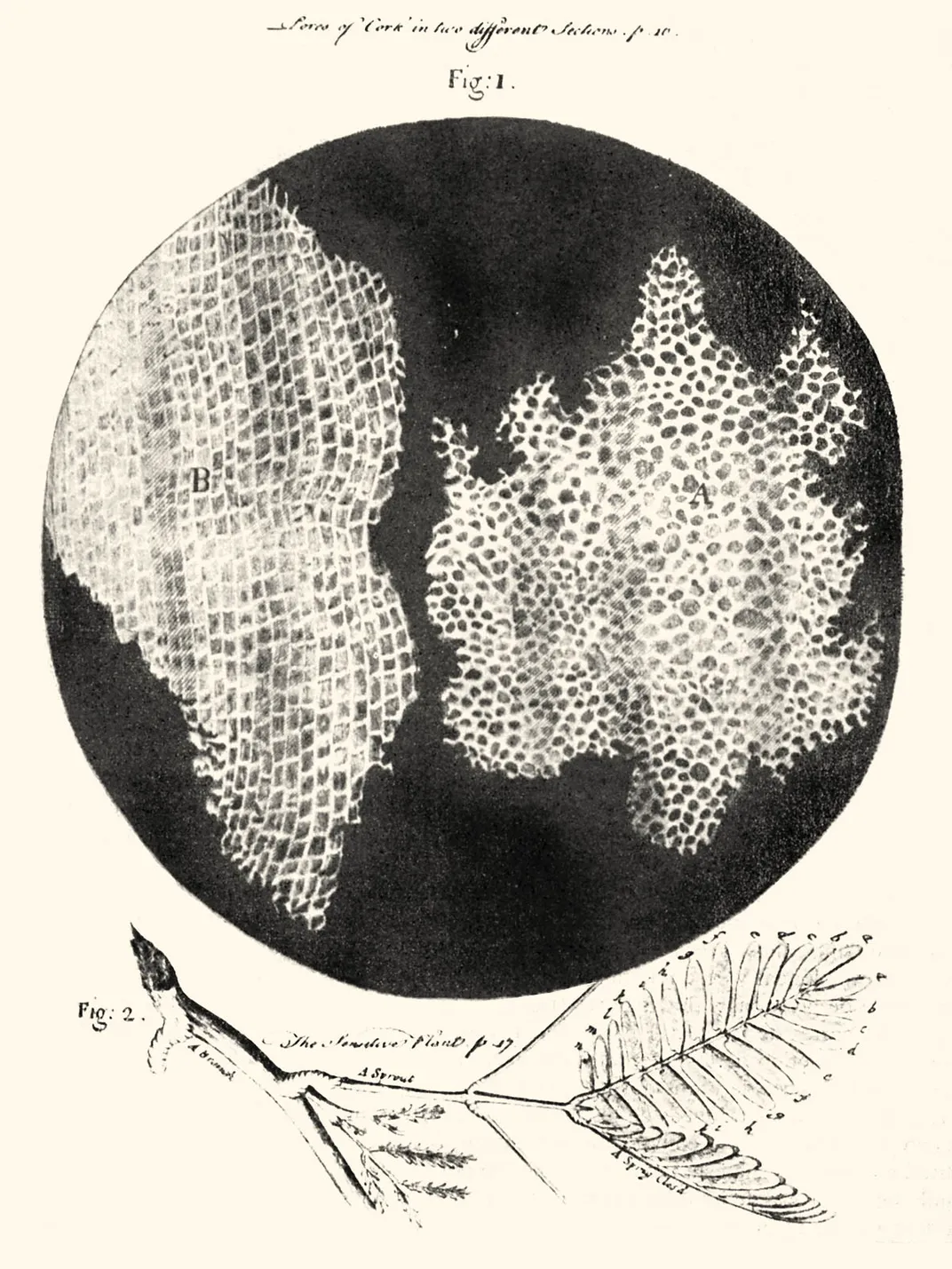
In 1676, Dutch cloth merchant-turned-scientist Antony van Leeuwenhoek further improved the microscope with the intent of looking at the cloth that he sold, but inadvertently made the groundbreaking discovery that bacteria exist. His accidental finding opened up the field of microbiology and the basis of modern medicine; nearly 200 years later, French scientist Louis Pasteur would determine that bacteria were the cause behind many illnesses (before that, many scientists believed in the miasma theory that rotten air and bad odors made us sick).
“It was huge,” says Kevin Eliceiri, a microscopist at the University of Wisconsin Madison, of the initial discovery of bacteria. “There was a lot of confusion about what made you sick. The idea that there are bacteria and things in the water was one of the greatest discoveries ever.”
The next year, in 1677, Leeuwenhoek made another hallmark discovery when he identified human sperm for the first time. A medical student had brought him the ejaculate of a gonorrhea patient to study under his microscope. Leeuwenhoek obliged, discovered tiny tailed animals, and went on to find the same wriggling “animalcules” in his own semen sample. He published these groundbreaking findings but, as was the case for bacteria, 200 years passed before scientists understood the true significance of the discovery.
By the late 1800s, a German scientist named Walther Flemming discovered cell division which, decades later, helped clarify how cancer grows—a finding that would have been impossible without microscopes.
“If you want to be able to target part of cell membrane or a tumor, you have to watch it,” says Eliceiri.
While the original microscopes that Hooke and Leeuwenhoek used may have had their limitations, their basic structure of two lenses connected by a tubes remained relevant for centuries, says Eliceiri. In the past 15 years, advancements in imaging have moved into new realms. In 2014, a team of German and American researchers won the Nobel Prize in Chemistry for a method called super-resolution fluorescence microscopy, so powerful we can now track single proteins as they develop within cells. This evolving method, made possible through an innovative technique that makes genes glow or “fluoresce”, has potential applications in combatting diseases such as Parkinson’s and Alzheimer’s.
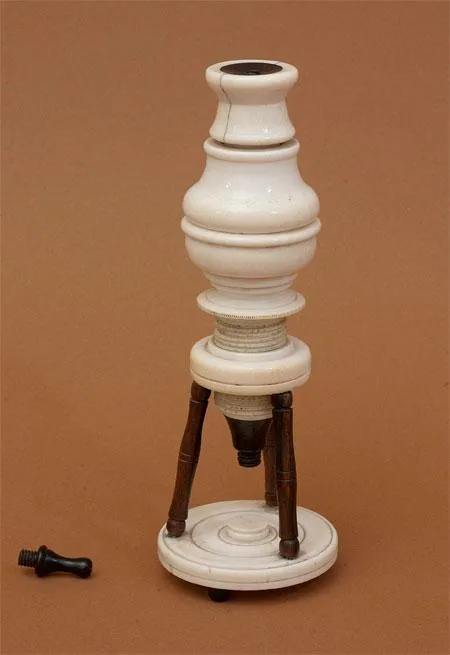
Ruzin heads the Biological Imaging Facility at the University of California at Berkeley, where researchers use the technology to explore everything from microstructures within the Giardia parasite and arrangements of proteins within bacteria. To help bring modern microscopy research into context, he makes a point of sharing some of the oldest items from the Golub Collection—one of the largest publicly displayed collections in the world, containing 164 antique microscopes dating back to the 17th century—with his undergraduate students. He even lets them handle some of the oldest in the collection, including an Italian one made of ivory around 1660.
“I say ‘don’t focus it because it will break,’ but I let students look through it, and it kinds of brings it home,” says Ruzin.
Still, despite the power of super-resolution microscopy, it does pose new challenges. For example, any time a specimen moves under high resolution, the image blurs, says Ruzin. “If a cell vibrates just by thermal motion, bouncing around by water molecules hitting it because they are warm, this will kill super resolution because it takes time,” says Ruzin. (For this reason, researchers don’t generally use super-resolution microscopy to study live samples.)
But technology like Amos’ Mesolens—with a much lower magnification of just 4x but a much wider field of view capable of capturing up to 5 mm, or about the width of a pinky fingernail—can image live specimen. This means they can watch a mouse embryo develop in real time, following genes associated with vascular disease in newborns as they become incorporated in the embryo. Prior to this, scientists would use X-rays to study vascular disease in embryos, but wouldn’t get detail down to the cellular level as they do with the Mesolens, Amos says.
“It’s almost unheard of for anyone to design a new objective lens for light microscopy and we have done this to try to accommodate the new types of specimens that biologists want to study,” says Amos’ colleague Gail McConnell at University of Strathclyde Glasgow, explaining that scientists are interested in studying intact organisms but don’t want to compromise the amount of detail they can see.
So far, the data storage industry has expressed interest in using the Mesolens to study semiconductor materials, and members of the oil industry have been interested in using it to image materials from prospective drilling sites. The lens design picks up light particularly well, allowing researchers to watch intricate details unfold such as cells in a metastasizing tumor migrating outward. But the true potential of these new techniques remains to be seen.
“If you develop an objective different than anything that has been made for the past 100 years, it opens up all kinds of unknown possibilities,” says Amos. “We are just beginning to get at what those possibilities are.”
Editor's Note, March 31, 2017: This post has been edited to reflect that Leeuwenhoek did not improve the compound microscope and that Ruzin's collection dates back to the 17th century.