Thirty-five years ago, a powerful drought shriveled crops in the American Midwest in the early spring. By summertime, 1988 was shaping up to be the hottest year on record.
That June 23, James Hansen, the director of NASA’s Institute for Space Studies and one of the nation’s leading climate scientists, clarified the looming threat. “It is,” he told the U.S. senators before him “time to stop waffling so much and say that the evidence is pretty strong that the greenhouse effect is here.” In the testimony before the Senate Energy and Natural Resources Committee, Hansen was joined by other prominent scientists also sounding the alarm. According to the New York Times’ reporting at the time, some senators called for “action now on a broad national and international program to slow the pace of global warming,” though that was far from the consensus opinion.
Hansen’s testimony to Congress that sweltering day marked the beginning of a slow but growing public understanding of the greenhouse effect, or climate change, as we term it today. Today, as reflected in a 2022 poll from the Pew Research Center, the majority of Americans recognize the reality of climate change, even if the history that brought us to this point isn’t fully understood.
As the anniversary of Hansen’s congressional testimony on June 23, 1988, approached, curators at the Smithsonian’s National Museum of American History decided to take a look at the museum’s existing collections to find that history.
Objects they identified provide insight into the push and pull inherent in the history of climate change. Americans have been building the tools that will help us to combat climate change for decades, even centuries. Unfortunately, they have also been building and using technologies that contribute to climate change.
Several objects illustrate how and why we have approached a tipping point in terms of the damaging effects climate change is having on our world. The North Atlantic is facing its warmest temperatures ever recorded, and heat waves in Mexico and India have killed untold amounts of wildlife in addition to more than 100 humans. Other objects illustrate the ways in which scientists have sought to understand the threat facing humanity and how we can best address this threat.
The original battery, 1799
/https://tf-cmsv2-smithsonianmag-media.s3.amazonaws.com/filer_public/e9/79/e979f9f1-b809-4b20-8109-23c21f0532b6/jn2023-00606-web.jpg)
Today, people are adopting batteries to power an ever-growing range of devices. No longer only for flashlights and portable electronics, batteries today power many cars and some homes.
They work by chemical action, generating electricity when charges pass from one material to another. This small copper disc, above, comes from the original battery made by Alessandro Volta of Italy at the turn of the 19th century.
Volta stacked alternating discs of metals such as copper and zinc between salt-water-soaked pads, creating what he called a “pile.” His battery produced a steady trickle of electricity. Researchers during the following two centuries came to understand how batteries work and created more powerful versions using a variety of materials.
Today, people depend on the descendants of Volta’s batteries as one tool in the effort to replace the fossil fuels that emit greenhouse gases. Nonetheless, care must be taken to avoid environmental pollution when making and disposing of batteries, and to use green power to recharge them. —Harold D. Wallace, curator of electricity, Division of Work and Industry
American felling ax, 19th century
/https://tf-cmsv2-smithsonianmag-media.s3.amazonaws.com/filer_public/41/36/41364e43-31c7-4913-b06b-073c36702793/ahb2017q006252.jpg)
Forests are one of our greatest defenses against climate change, which means their destruction makes any mitigation efforts acutely more difficult.
Human beings have relied on trees for construction, fuel and wood-derived products for ages, and axes have greatly aided people’s ability to harvest this natural resource. However, as populations have grown over time—and, with them, consumerist appetites—deforestation has occurred more rapidly and on larger scales. The impact of deforestation ranges from the immediate and most tangible, such as the loss of habitat, to the distant and least perceptible, such as threatening climate regulation. Forests support the stability of our climate in two related ways: Trees remove carbon dioxide from the atmosphere by absorbing this greenhouse gas, and they store great quantities of carbon in their branches and trunks.
Although deforestation is not new, felling axes had limitations as tools, which meant the rate of harvesting timber with these axes was relatively slow, especially in places like colonial and antebellum America. Now, large swaths of forest that are centuries old can be cleared in months, if not weeks. While it would be easy to point to technological innovation as a prime contributor to deforestation, the main culprits are consumers and our seemingly insatiable demand for convenience and comfort. Such demand is the reason why agriculture is one of the biggest drivers of deforestation today. To change this requires a change in global production systems, specifically those related to everyday foods we eat and products we use. —Tony C. Perry, curator of environmental history, Division of Work and Industry
Kerosene lamp, 1876
/https://tf-cmsv2-smithsonianmag-media.s3.amazonaws.com/filer_public/88/4c/884c547a-0a33-4507-a41a-dbaa30a5cb49/jn2014-4155.jpg)
The quest to conquer the night with artificial light has had long-term consequences. In the 1800s, lighting systems shifted from candles to whale oil, then camphene (turpentine and alcohol), and then kerosene (patented in 1854). While kerosene flames appear smokeless, they emit invisible greenhouse gases.
Oil lamps like the one patented by John Irwin in 1862 revolutionized society. Bright and economical artificial light changed concepts of time, work, leisure activities and consumption. The demand for kerosene was gigantic. Companies drilled for petroleum, from which kerosene is made, in the United States, Azerbaijan, Indonesia and beyond. U.S. domestic crude oil production skyrocketed—1,000 barrels in 1860; 14,000 barrels in 1870; 72,000 barrels in 1880; and 126,000 barrels in 1890. In the 1870s, kerosene was America’s fourth-largest export, with oil exports accounting for half of U.S. oil production.
In the late 1880s, demand for kerosene for lamps began to drop as electric lights became a popular alternative. The addiction to petroleum continued, however, as other uses such as gasoline fueled even greater demand. —Peter Liebhold, emeritus curator, Division of Work and Industry
Medicine and science apparatus for administering nitrous oxide and other anesthetic gases, circa 1890
Since its introduction as an anesthetic in the 19th century, nitrous oxide (or laughing gas) has enabled many of us to put aside our fears and visit the dentist. But nitrous oxide has not come without cost. Because it is about 300 times more potent as a greenhouse gas than carbon dioxide, its use plays an outsized role in damaging the planet. Nitrous oxide also lingers longer in the atmosphere than carbon dioxide.
In 1884, 40 years after Horace Wells first demonstrated that nitrous oxide could relieve pain, Amos Long, a Michigan dentist, patented this gasometer. At the turn of the 20th century, Long’s invention, with its elaborate decorations, was commonly found in dentists’ offices. The apparatus has two tanks, the smaller of which held a canister of compressed nitrous oxide. The gas was then released into the larger tank and delivered to the patient via the rubber tubing and mask shown here. Although most commonly used in dentistry, nitrous oxide was also given to women in labor.
Today, the health care industry is responsible for approximately 8.5 percent of all greenhouse gases in the United States. Within this industry, anesthesia such as nitrous oxide contributes just over 50 percent of all operating room greenhouse gas emissions. Yet few of us realize that that our expectations of a pain-free dentist visit are linked to the long history of climate change. —Alexandra M. Lord, chair and curator of medicine, Division of Medicine and Science
Photosynthometer, circa 1905
Together, this pear-shaped plant chamber and graduated cylinder enabled college students to quantify the absorption of carbon dioxide and release of oxygen by a small plant. Botanist and college professor William Francis Ganong first described this apparatus in 1905, and by that point the gaseous exchange of photosynthesis was well known. The biological mechanism responsible for the process that combines water, carbon dioxide and solar energy to produce sugar and oxygen was not.
/https://tf-cmsv2-smithsonianmag-media.s3.amazonaws.com/filer_public/74/cc/74cc3ed1-afa5-4c9a-b96d-e92b6e2d4893/jn2023-00590-web.jpg)
This photosynthometer hints at an increasing influence of chemistry—represented by the glassware—into the realm of botany. Decades of research and a host of scientists from multiple disciplines were needed to trace the path of carbon in photosynthesis. And that is but one small element of a larger complex cycle that takes carbon from the atmosphere to the earth and back to the atmosphere again. —Kristen Frederick-Frost, curator of modern science, Division of Medicine and Science
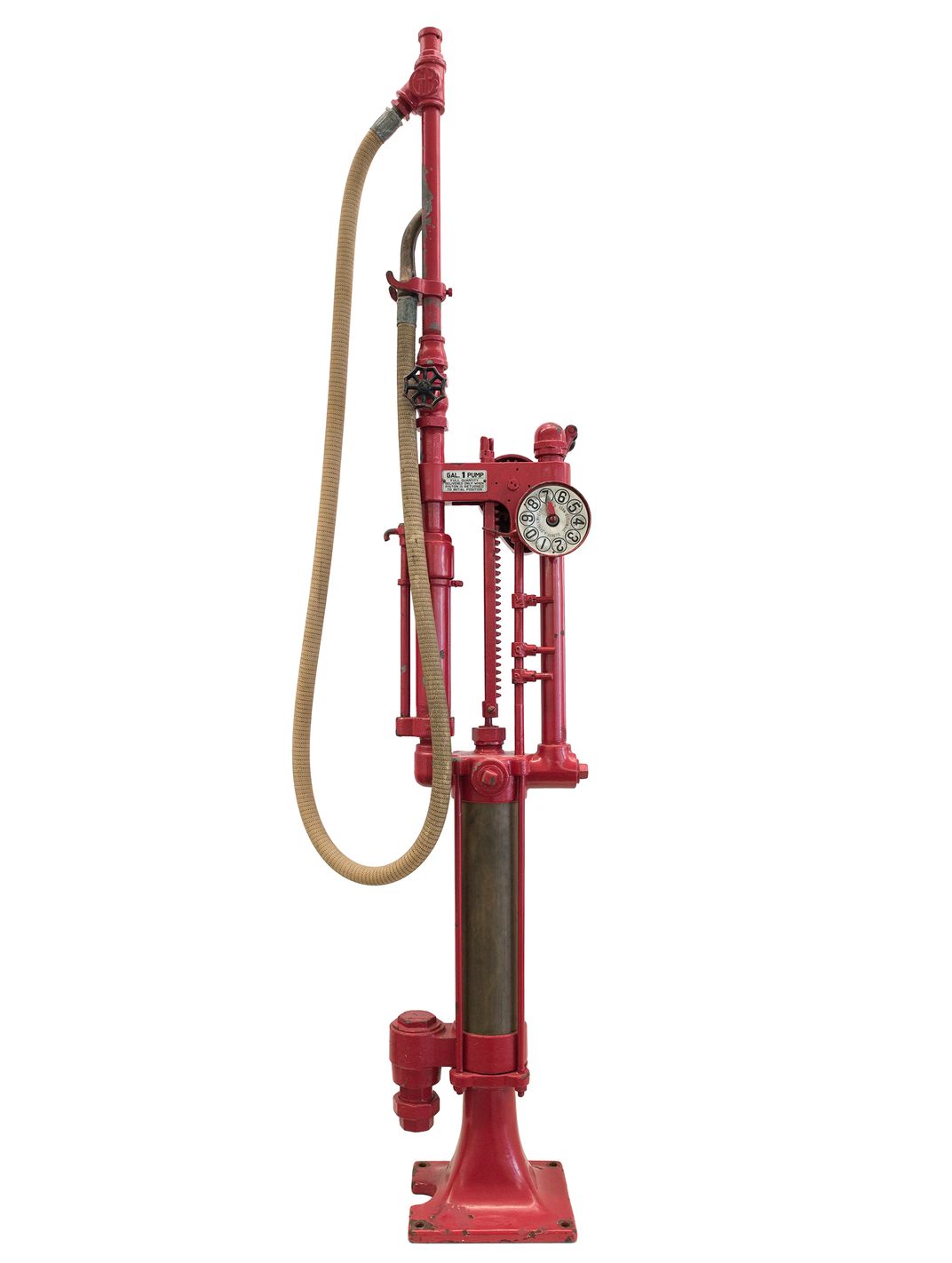
Gasoline pump, 1911
For over 100 years, the gasoline pump has symbolized the changing relationships among consumers, the oil industry, government and the environment. Charles Gilbert and John Barker formed a company in 1865 to make machinery for gas lighting systems. The popularity of automobiles increased demand for gasoline, even as other early automobiles used steam or electricity for fuel. In 1910, the Gilbert & Barker company added hand-operated gasoline pumps. Rather than selling gasoline from central depots, entrepreneurs built readily accessible consumer outlets, and the modern gas station was born. This pump, manufactured in 1911, is one of the first of its kind that counted the number of gallons delivered.
What climate change means for the future of gas pumps is unclear. With a relatively short range, electric vehicles need public recharging points. Unlike gas pumps, recharging stations do not have to be connected to large underground tanks and can be located anywhere that electricity is available. The use of public transportation might also move consumers away from individually operated vehicles. —P.L.
“Swift’s Premium Corned Beef” crate label, 1920s
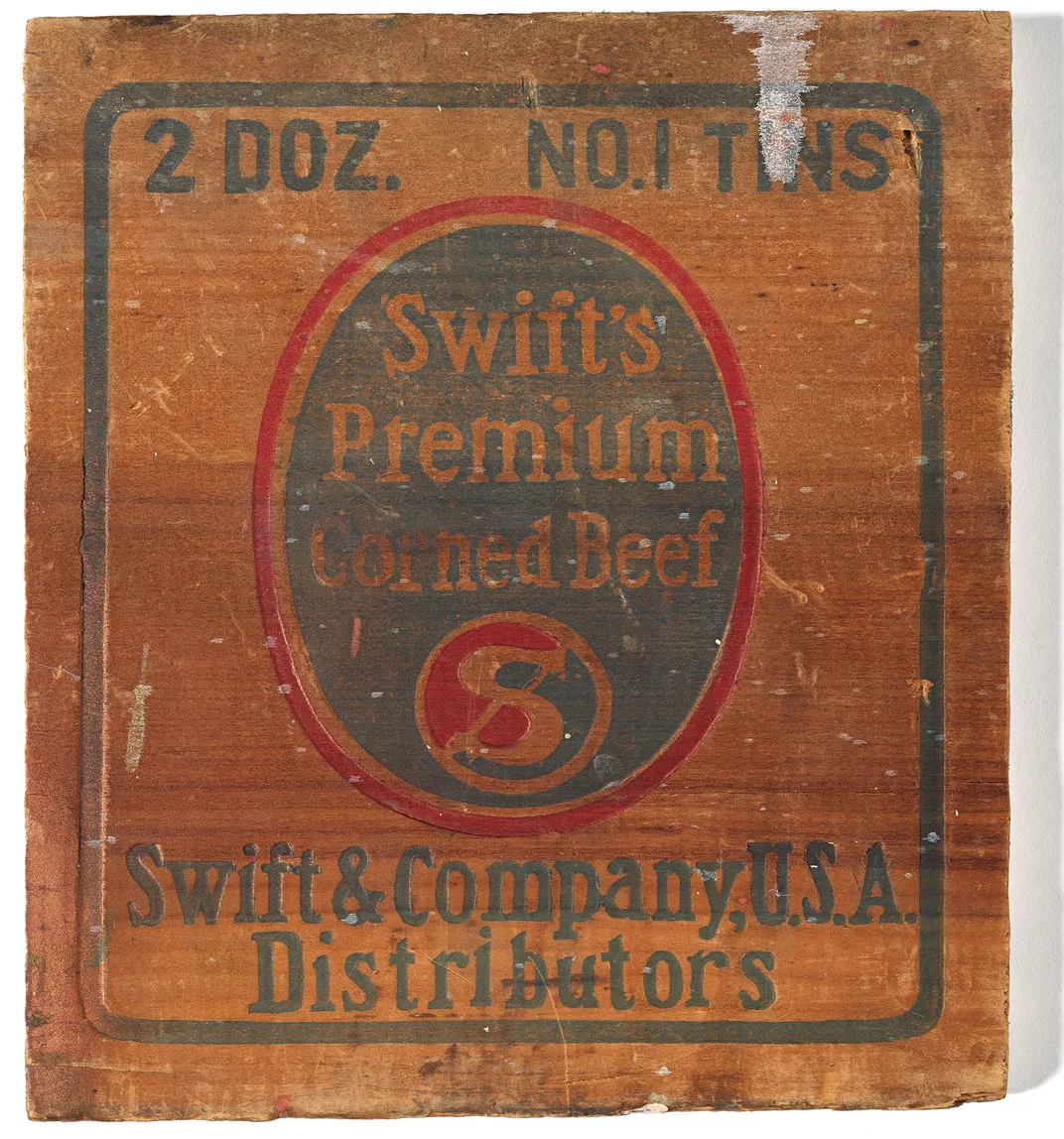
Since 2014, the United Nations has repeatedly confirmed that the greenhouse gas emissions from animal agriculture rival that of the transportation sector. The cattle industry specifically (including both dairy and beef) is responsible for more than 50 percent of the livestock sector’s impact on climate change. The roots of this tremendous carbon footprint have been traced to the adoption of beef grading in the mid-20th century and the ensuing rise of industrial feedlots, first in the U.S. and then around the world.
This Swift & Company crate label, titled “Swift’s Premium Corned Beef,” represents an early iteration of graded beef marketing. In the late 1920s, Swift & Company, followed by other meatpackers, began grading and marking beef using their own brand names, such as Swift Premium, instead of USDA Choice. Eventually, with the support of Corn Belt cattle breeders, the Department of Agriculture’s national beef grading standards won market dominance. The USDA grading system was unequivocal in its prescription to cattle farmers that grain-fed cattle produced superior beef to grass-fed animals. Thus, producers were pushed into intensifying production through concentrated corn-feeding at industrial feedlots, leading to an explosion of the cattle population and an exponential rise in carbon emissions by the 1970s. —Abeer Saha, curator, Division of Work and Industry
/https://tf-cmsv2-smithsonianmag-media.s3.amazonaws.com/filer_public/b1/9c/b19c5f22-4cb2-484e-a41b-9eddff412732/et2012-13752.jpg)
Caterpillar diesel engine “Old Betsy,” 1930
“Old Betsy” is the nickname for Caterpillar’s first diesel engine model, the D9900, built in 1930. It is one of the earliest mass-produced diesel engines ever made in the U.S. The four-cylinder engine uses various fuels and generates 86.8 horsepower at 700 revolutions per minute. These engines were used to drive heavy machinery and earth-moving equipment in the construction and agricultural industries. Manufacturers of such heavy equipment preferred transitioning from gasoline to diesel, in part because the heavier classes of motor fuel offered efficiencies.
Today, diesel engines account for over a quarter of the U.S. transportation sector’s carbon dioxide emissions. Straightforward comparisons of the carbon footprint between diesel and petrol vehicles are difficult because even though diesel fuels contain more carbon, they can burn more efficiently than petroleum. This means that a diesel vehicle may release less carbon dioxide than a petroleum vehicle traveling the same distance. This, however, varies from vehicle to vehicle, and design to design. An important argument against diesel engines is that they are also a major source of nitrogen oxide emissions and particulate matter, which can have serious respiratory and cardiovascular effects, as well as severe global warming potential. —A.S.
Deep-sea thermometer, circa 1940
/https://tf-cmsv2-smithsonianmag-media.s3.amazonaws.com/filer_public/12/f7/12f72c41-6036-4c90-adbb-35594135ab18/jn2023-00592-web.jpg)
When we think of “climate change,” what generally comes to mind is rising temperatures: warming of the atmosphere that is expected to lead to more severe storms, changes in rainfall patterns, environmental degradation and much more. The evidence for these rising temperatures comes through weather records, measured by thermometers all over the world and preserved by meteorological offices. Valuable though these records are, however, they tell only part of the story, because the atmosphere is not the only part of the earth that takes in heat from the sun and sends it out again into space—the oceans cover three-quarters of the surface of our planet.
Scientists have been aware of the importance of the oceans in determining climate since the 17th century, but for obvious reasons collecting the relevant data has not been easy. It has been difficult enough—and expensive—for governments to send out oceanographic expeditions to investigate the physical properties of the surface waters of the ocean, but it is far harder to learn about what goes on in the depths, often miles down. These deep masses of water, at temperatures barely above freezing, circulate slowly, transporting equatorial warmth to the polar seas. Differences of small fractions of a degree can be highly significant. But how do you take a reading of a thermometer hundreds of fathoms down?
Instrument makers have devised a number of ingenious solutions to this problem; this thermometer embodies several of them in a combination that was standard from about 1890 until 1970, when it was finally superseded by modern electronic methods. First, to eliminate the effect of the immense pressure of the water on the bulb of the thermometer, it was encased in a strong external tube. Then, to preserve the reading recorded at the desired depth, the instrument is designed so that when turned upside-down, the mercury column separates at a defined point. After being hauled up from the depths, it is read at leisure from the inverted scale.
This reversing principle was introduced by the London firm Negretti & Zambra, makers of optical and meteorological instruments, in 1874. The thermometer is mounted in a special frame attached to the sounding line; a “messenger” weight slides down the line and releases the reversing mechanism that turns the thermometer over. It also releases another messenger below the thermometer, which continues on down to the next thermometer in the series, and so on. Attached to each reversing frame, in addition to the thermometer, is a “Nansen bottle,” a special container that closes when reversed, securing a sample of the water at that depth for analysis. —Roger Sherman, curator of modern physics, Division of Medicine and Science
/https://tf-cmsv2-smithsonianmag-media.s3.amazonaws.com/filer_public/52/6e/526e9eeb-cbd1-42f7-8339-cfa607f49736/jn2023-00588-web.jpg)
Keeling flask, late 20th-century
A hissing noise escaped from this flask as it was opened at an altitude of more than 11,000 feet at the Mauna Loa Observatory in Hawaii. The vacuum created in its interior was replaced by a big gulp of air from the North Pacific. Resealed, the glass flask returned to the Scripps Institution of Oceanography at the University of California San Diego—a trip it made many times before, as had similar flasks in preceding years. The remote sampling conducted with these flasks paired with continuous measurements made at the observatory enabled the creation of an atmospheric carbon dioxide data set that stretched from the 1958 to the present.
Scientist Charles David Keeling first recognized that his data demonstrated a seasonal variation that corresponded with plant growth, but time added another finding: a steadily rising carbon dioxide background due to our consumption of fossil fuels. The Keeling Curve became an icon of climate science and a call to action, as the overall rise in this critical greenhouse gas made the threat of global warming manifest for many. In a 2005 obituary for Keeling in the New York Times, James Hansen summed up the import of Keeling’s work, “He altered our perspectives about the degree to which the earth can absorb the human assault.” —K.F.
Solarex “Solar Energizer” solar panel, circa 1983
The history of climate change is rife with contradictions. Did you know that the petroleum industry played a significant role in the commercialization of solar panels? This Solarex “Solar Energizer” powered equipment on an offshore oil rig.
/https://tf-cmsv2-smithsonianmag-media.s3.amazonaws.com/filer_public/29/1b/291b1a87-9193-4f35-b997-212d5aa2de02/jn2023-00605-web.jpg)
Scientists in the 19th century discovered that sunlight could generate electricity in certain materials. In 1954, a team at Bell Telephone Laboratories invented a practical solar cell. The company used solar cells to power radio and telephone transmitters in remote locations where it was too expensive or impossible to run electric power lines, such as on mountain peaks and on satellites.
By the early 1980s, the oil industry invested in solar panel research because it needed to solve two problems, one offshore and one onshore. Oil companies used short-lived, heavy batteries to power navigation beacons and lights on offshore drilling platforms. Onshore, the companies needed small currents of electricity to slow corrosion of oil well casings that passed through salty groundwater.
Solar panels cost less than hauling batteries offshore or running power lines to remote oil fields. Oil industry purchases lowered costs further, while proving that solar cells were not just for use in space. Solar panels now generate an increasing share of the world’s electricity, helping us move away from fossil fuels. —H.W.
/https://tf-cmsv2-smithsonianmag-media.s3.amazonaws.com/filer_public/00/58/00581b69-4a4b-444f-8775-b0c543a4c4be/jn2014-3858.jpg)
Levee wall fragment, 2005
One of the great ironies of climate change is the expectation that scientists and engineers will solve the problems that arguably scientists and engineers helped cause.
People have long located cities near streams, rivers, lakes and oceans. These locations come with rewards—beauty, water supply and transportation. But, historically, being near water also increases the potential of floods. Climate change leads to rising sea levels and more intense weather events. Experts don’t believe climate change is causing a greater number of hurricanes and cyclones, but they think the same number of storms are dumping more rain, have higher wind speeds and create larger storm surge.
In 2005, wind-driven water from Hurricane Katrina breached the flood walls atop the London Avenue Canal levee, and large portions of New Orleans flooded. An investigation of the disaster found an engineering error in the flood wall foundation design. The old wall was replaced with a more robust structure. Who will win in the future—engineering or nature? —P.L.
Container ship model, 2006
/https://tf-cmsv2-smithsonianmag-media.s3.amazonaws.com/filer_public/ac/01/ac01a49e-3a0c-49cd-9332-c1c12fee99f5/2003-27979.jpg)
The history of container ships provides an opportunity for insight into the complexities of solving climate change. Containers, 20- or 40-foot steel boxes carried on ships, trains and trucks, revolutionized manufacturing by making foreign production cost effective. On the one hand these oceanic behemoths powered by monstrous engines contribute about 3 percent of air pollution. Less considered is the climate impact of consumer demand for cheap goods being transported by the ships. Solutions to the transportation of goods problem are not always obvious.
The Emma Maersk is gigantic and efficient. Launched in 2006, the ship is 1,302 feet long and 183 feet wide, and it can carry about 11,000 20-foot containers. The Emma Maersk is powered by a 14-cylinder diesel engine that produces 109,000 horsepower and consumes, when underway, 3,600 gallons of heavy bunker fuel per hour. At the time of its construction the Emma Maersk was the largest container ship in the world.
In the 1700s, most goods were produced locally, but the Industrial Revolution created efficiencies that encouraged national and global consumption. Today, manufacturers produce goods and ship them around the world to meet consumers’ voracious demand. Is cheap transportation feeding climate change, or should we look to ourselves and consider that our demand of goods is a driving force in climate change? —P.L.
Mosquito nets, 2017
/https://tf-cmsv2-smithsonianmag-media.s3.amazonaws.com/filer_public/e1/70/e1709bb6-58d4-49e2-afef-7a317758c79c/jn2023-00613-web.jpg)
Weather events become more destructive when mixed with climate change and colonialism. The line connecting those histories may not be immediately evident, but the disastrous result is clear, as Hurricane Maria demonstrated in 2017.
Puerto Rico, with a colonial history going back 500 years, was devastated by Hurricane Maria. The massive size and force of the storm caused over 4,000 deaths, countless injuries and lingering trauma.
Converging legacies amplified the damage. The energy systems upon which Puerto Rico and the world depend are not sustainable, and the places constrained by colonialist practices struggle the most. In Puerto Rico, industrialization, the debt crisis and other extractive operations left a precarious infrastructure that did not adequately meet the needs of people living in a tropical environment.
People who relied upon air conditioning or the trade winds for cooling found their homes breached or destroyed, with roofs and windows blown away. Energy dependency and destruction of screens and other barriers allowed mosquitos to feast around the clock. Environmentalist Fernando Silva observed the misery of sleep deprivation and connected it to the mosquitoes. He began a project with several women to help people craft handmade mosquito nets for their neighbors, rescuing an art that had been lost with the introduction of air conditioning.
—Katherine Ott, curator of medicine, Division of Medicine and Science
Bitcoin ring, 2018
Coins and banknotes have been the predominate forms of circulating money for centuries. Their production relies on extractive industries, such as the mining of metals for coins. On the surface, digital currencies would appear to be a more sustainable alternative. Many cryptocurrencies, such as Bitcoin, however, involve a digital mining process that requires large amount of electricity to run cryptographic code. Globally, a majority of electricity is generated through the use of fossil fuels.
/https://tf-cmsv2-smithsonianmag-media.s3.amazonaws.com/filer_public/8a/2d/8a2d781b-7586-457e-85f6-00ad5c5d721a/jn2023-00621.jpg)
Bitcoin is the most widely used cryptocurrency among the more than 20,000 that have emerged since 2009. It is part of popular culture and has come to symbolize a rejection of centralized power and conventional practices. One clever example of this is the Bitcoin ring. An alternative to the traditional diamond engagement ring, it shifts the money that might have been spent on a diamond into an investment in Bitcoin. The 3D-printed QR code on the ring links to the blockchain and shows how much Bitcoin the owner holds. While the environmental impact is not as visible as the extractive practices of diamond mining, the Bitcoin ring may not be as environmentally friendly as it appears. —Ellen Feingold, curator of the national numismatic collection, Division of Work and Industry